The Google team uses a row of nine solid-state qubits, fashioned from cross-shaped films of aluminium about 400 micrometres from tip to tip. These are deposited onto a sapphire surface. The researchers cool the aluminium to 0.02 degrees kelvin, turning the metal into a superconductor with no electrical resistance. Information can then be encoded into the qubits in their superconducting state.
The interactions between neighboring qubits are controlled by ‘logic gates’ that steer the qubits digitally into a state that encodes the solution to a problem. As a demonstration, the researchers instructed their array to simulate a row of magnetic atoms with coupled spin states — a problem thoroughly explored in condensed-matter physics. They could then look at the qubits to determine the lowest-energy collective state of the spins that the atoms represented.
This is a fairly simple problem for a classical computer to solve. But the new Google device can also handle so-called ‘non-stoquastic’ problems, which classical computers cannot. These include simulations of the interactions between many electrons, which are needed for accurate computer simulations in chemistry. The ability to simulate molecules and materials at the quantum level could be one of the most valuable applications of quantum computing.
This new approach should enable a computer with quantum error correction, says Lidar.
“With error correction, our approach becomes a general-purpose algorithm that is, in principle, scalable to an arbitrarily large quantum computer,” says Alireza Shabani, another member of the Google team.
The Google device is still very much a prototype. But Lidar says that in a couple of years, devices with more than 40 qubits could become a reality.
“At that point,” he says, “it will become possible to simulate quantum dynamics that is inaccessible on classical hardware, which will mark the advent of ‘quantum supremacy’.”
Spin-chain problem and device. a, We implement one-dimensional spin problems with variable local fields and couplings between adjacent spins. An example of a stoquastic problem Hamiltonian with local x and z fields, indicated by the gold arrows in the spheres, and σzσz couplings, whose strength is indicated by the radius of the links, is shown. Red denotes a ferromagnetic (J = +1) and blue an antiferromagnetic (J = −1) link. The problem Hamiltonian is for the instance shown in c. b, Optical picture of the superconducting quantum device with nine Xmon22 qubits Q0–Q8 (false-coloured cross-shaped structures), made from aluminium (light) on a sapphire substrate (dark). Connections to read-out resonators are at the top; control wiring is at the bottom. Scale bar, 200 μm.
Dwave Chip which has no error correction by 1000-2000 qubits
Nature – Digitized adiabatic quantum computing with a superconducting circuit
Quantum mechanics can help to solve complex problems in physics and chemistry, provided they can be programmed in a physical device. In adiabatic quantum computing, a system is slowly evolved from the ground state of a simple initial Hamiltonian to a final Hamiltonian that encodes a computational problem. The appeal of this approach lies in the combination of simplicity and generality; in principle, any problem can be encoded. In practice, applications are restricted by limited connectivity, available interactions and noise. A complementary approach is digital quantum computing6, which enables the construction of arbitrary interactions and is compatible with error correction7,8, but uses quantum circuit algorithms that are problem-specific. Here we combine the advantages of both approaches by implementing digitized adiabatic quantum computing in a superconducting system. We tomographically probe the system during the digitized evolution and explore the scaling of errors with system size. We then let the full system find the solution to random instances of the one-dimensional Ising problem as well as problem Hamiltonians that involve more complex interactions. This digital quantum simulation of the adiabatic algorithm consists of up to nine qubits and up to 1,000 quantum logic gates. The demonstration of digitized adiabatic quantum computing in the solid state opens a path to synthesizing long-range correlations and solving complex computational problems. When combined with fault-tolerance, our approach becomes a general-purpose algorithm that is scalable
SOURCES – Nature
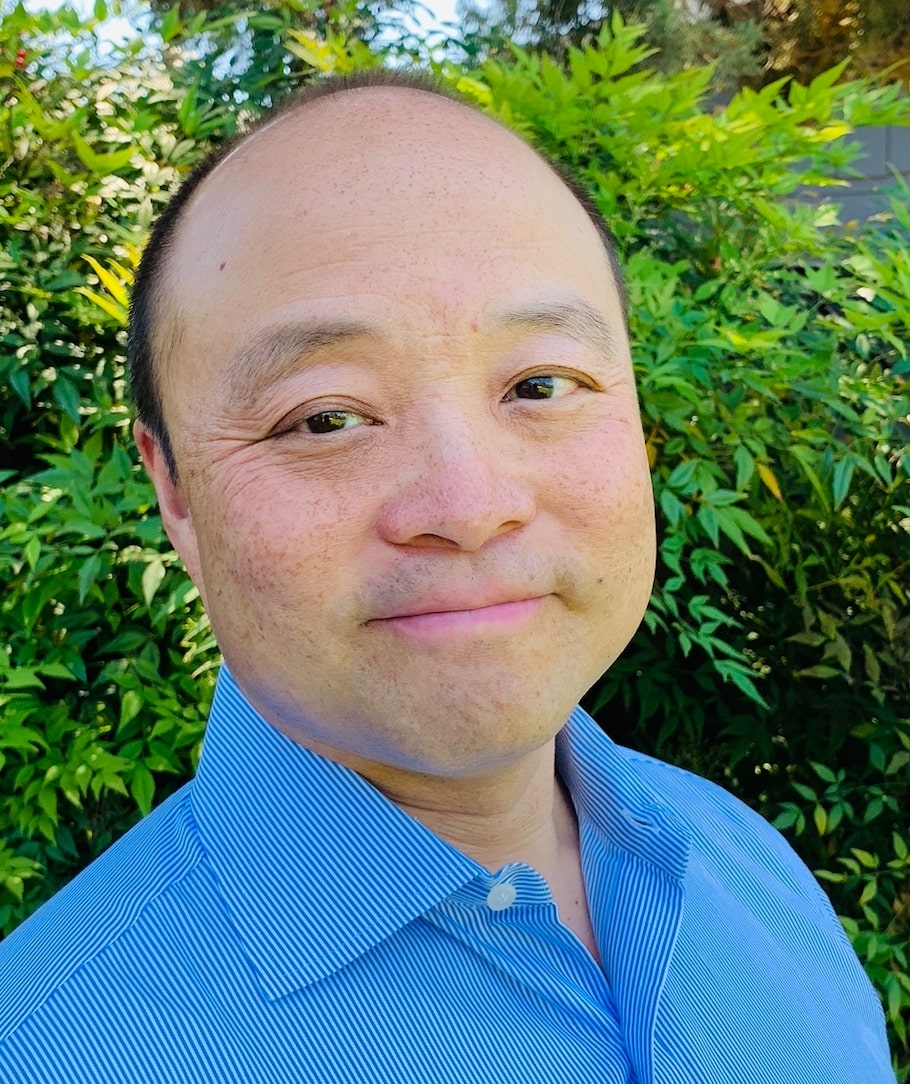
Brian Wang is a Futurist Thought Leader and a popular Science blogger with 1 million readers per month. His blog Nextbigfuture.com is ranked #1 Science News Blog. It covers many disruptive technology and trends including Space, Robotics, Artificial Intelligence, Medicine, Anti-aging Biotechnology, and Nanotechnology.
Known for identifying cutting edge technologies, he is currently a Co-Founder of a startup and fundraiser for high potential early-stage companies. He is the Head of Research for Allocations for deep technology investments and an Angel Investor at Space Angels.
A frequent speaker at corporations, he has been a TEDx speaker, a Singularity University speaker and guest at numerous interviews for radio and podcasts. He is open to public speaking and advising engagements.