High-pressure researchers, including Carnegie’s Ho-kwang (Dave) Mao, have now modeled three hydrogen-dense metal alloys and found there are pressure and temperature trends associated with the superconducting state—a huge boost in the understanding of how this abundant material could be harnessed. Computer modeling indicates that superconductivity for trihydrides (Hydrogen compounds with 3 hydrogens) set in at pressures between roughly 100,000 to 200,000 times atmospheric pressure at sea level (10 to 20 GPa), which is an order of magnitude lower (ten times less) than the pressures for related compounds that bind with four hydrogens instead of three. the hope has been that metallic superconducting hydrogen could be a room temperature superconductor.
Physicists have long wondered whether hydrogen, the most abundant element in the universe, could be transformed into a metal and possibly even a superconductor—the elusive state in which electrons can flow without resistance. They have speculated that under certain pressure and temperature conditions hydrogen could be squeezed into a metal and possibly even a superconductor, but proving it experimentally has been difficult.
Scientists have found that in addition to chemical manipulation to raise the transition temperature, superconductivity can also be induced by high pressure. Theoretical modeling is very helpful in defining the characteristics and pressures that can lead to high transition temperatures. In this study, the scientists modeled basic properties from first principles—the study of behavior at the atomic level—of three metal hydrides under specific temperature, pressure, and composition scenarios. Metal hydrides are compounds in which metals bind to an abundance of hydrogen in a lattice structure. The compounds were scandium trihydride (ScH
3),yttrium trihydride (YH3) and lanthanum trihydride (LaH3).
“We found that superconductivity set in at pressures between roughly 100,000 to 200,000 times atmospheric pressure at sea level (10 to 20 GPa), which is an order of magnitude lower than the pressures for related compounds that bind with four hydrogens instead of three,” remarked Mao, of Carnegie’s Geophysical Laboratory. Lanthanum trihydride stabilized at about 100,000 atmospheres and a transition temperature of – 423°F (20 Kelvin), while the other two stabilized at about 200,000 atmospheres and temperatures of -427 °F (18 K) and -387 °F (40 K) for ScH3 and YH3 respectively.
PNAS- General trend for pressurized superconducting hydrogen-dense materials
The long-standing prediction that hydrogen can assume a metallic state under high pressure, combined with arguments put forward more recently that this state might even be superconducting up to high temperatures, continues to spur tremendous research activities toward the experimental realization of metallic hydrogen. These efforts have however so far been impeded by the enormous challenges associated with the exceedingly large required pressure. Hydrogen-dense materials, of the MH4 form (where M can be, e.g., Si, Ge, or Sn) or of the MH3 form (with M being, e.g., Al, Sc, Y, or La), allow for the rather exciting opportunity to carry out a proxy study of metallic hydrogen and associated high-temperature superconductivity at pressures within the reach of current techniques. At least one experimental report indicates that a superconducting state might have been observed already in SiH4, and several theoretical studies have predicted superconductivity in pressurized hydrogen-rich materials; however, no systematic dependence on the applied pressure has yet been identified so far. In the present work, we have used first-principles methods in an attempt to predict the superconducting critical temperature (Tc) as a function of pressure (P) for three metal-hydride systems of the MH3 form, namely ScH3, YH3, and LaH3. By comparing the obtained results, we are able to point out a general trend in the Tc-dependence on P. These gained insights presented here are likely to stimulate further theoretical studies of metallic phases of hydrogen-dense materials and should lead to new experimental investigations of their superconducting properties.
The researchers also found that two of the compounds, LaH3 and YH3, had more similar distributions of vibrational energy to each other than to ScH3 at the superconducting threshold and that the transition temperature was highest at the point when a structural transformation occurred in all three. This result suggests that the superconducting state comes from the interaction of electrons with vibrational energy through the lattice. At pressures higher than 350,000 atmospheres (35 GPa) superconductivity disappeared and all three compounds became normal metals. In yttrium trihydride, the superconductivity state reappeared at about 500,000 atmospheres, but not in the others. The scientists attributed that effect to its different mass.
“The fact that the models predicted distinctive trends in the behavior for these three related compounds at similar temperatures and pressures is very exciting for the field,” commented Mao. “Previous to this study, the focus has been on compounds with four hydrogens. The fact that superconductivity is induced at lower pressures in the trihydrides makes them potentially more promising materials with which to work. The temperature and pressures ranges are easily attainable in the lab and we hope to see a flurry of experiments to bear out these results.” The team at Carnegie has embarked on their own experiments on this class of trihydrides to test these models.
OTHER NEW SUPERCONDUCTING RESEARCH
1. PNAS – High-pressure crystal structures and superconductivity of Stannane (SnH4)
There is great interest in the exploration of hydrogen-rich compounds upon strong compression where they can become superconductors. Stannane (SnH4) has been proposed to be a potential high-temperature superconductor under pressure, but its high-pressure crystal structures, fundamental for the understanding of superconductivity, remain unsolved. Using an ab initio evolutionary algorithm for crystal structure prediction, we propose the existence of two unique high-pressure metallic phases having space groups Ama2 and P63/mmc, which both contain hexagonal layers of Sn atoms and semimolecular (perhydride) H2 units. Enthalpy calculations reveal that the Ama2 and P63/mmc structures are stable at 96–180 GPa and above 180 GPa, respectively, while below 96 GPa SnH4 is unstable with respect to elemental decomposition. The application of the Allen-Dynes modified McMillan equation reveals high superconducting temperatures of 15–22 K for the Ama2 phase at 120 GPa and 52–62 K for the P63/mmc phase at 200 GPa.
2. PNAS – Percolative theories of strongly disordered ceramic high-temperature superconductors
Optimally doped ceramic superconductors (cuprates, pnictides, etc.) exhibit transition temperatures Tc much larger than strongly coupled metallic superconductors like Pb (Tc = 7.2 K, Eg/kTc = 4.5) and exhibit many universal features that appear to contradict the Bardeen, Cooper, and Schrieffer theory of superconductivity based on attractive electron-phonon pairing interactions. These complex materials are strongly disordered and contain several competing nanophases that cannot be described effectively by parameterized Hamiltonian models, yet their phase diagrams also exhibit many universal features in both the normal and superconductive states. Here we review the rapidly growing body of experimental results that suggest that these anomalously universal features are the result of marginal stabilities of the ceramic electronic and lattice structures. These dual marginal stabilities favor both electronic percolation of a dopant network and rigidity percolation of the deformed lattice network. This “double percolation” model has previously explained many features of the normal-state transport properties of these materials and is the only theory that has successfully predicted strict lowest upper bounds for Tc in the cuprate and pnictide families. Here it is extended to include Coulomb correlations and percolative band narrowing, as well as an angular energy gap equation, which rationalizes angularly averaged gap/Tc ratios, and shows that these are similar to those of conventional strongly coupled superconductors.
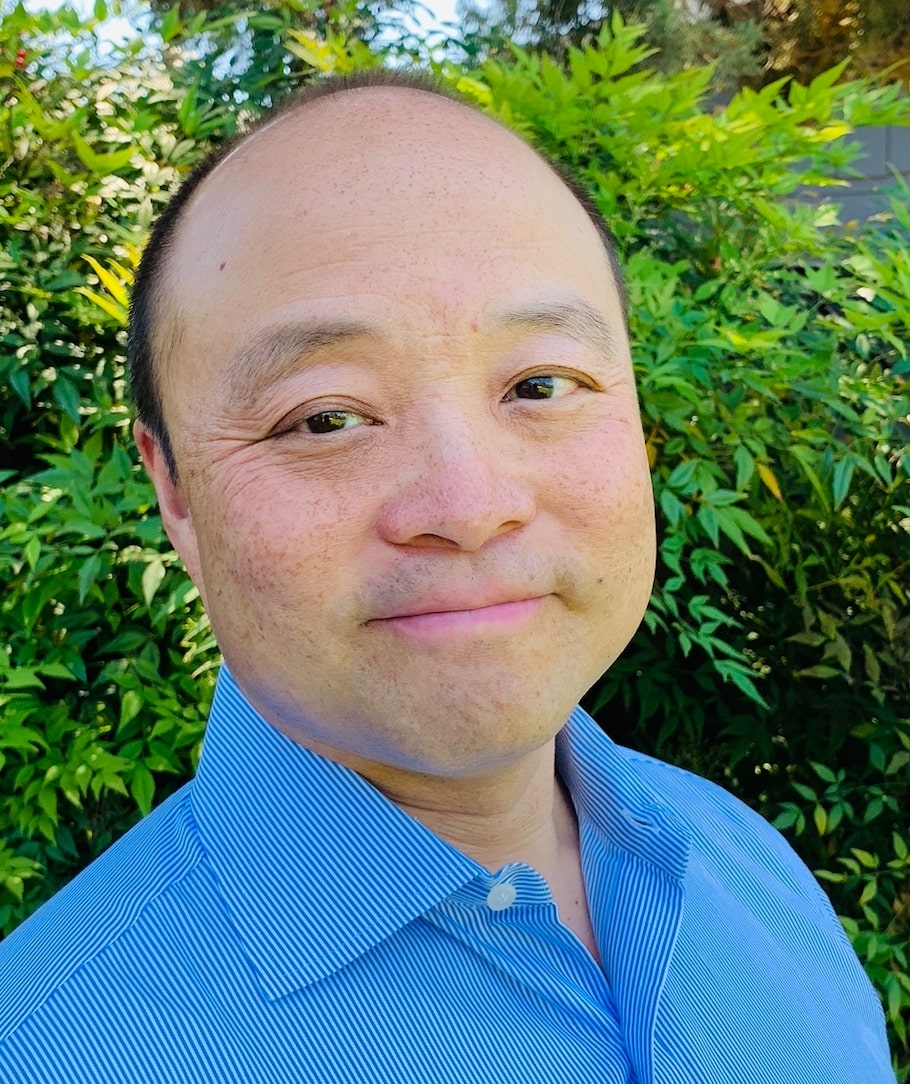
Brian Wang is a Futurist Thought Leader and a popular Science blogger with 1 million readers per month. His blog Nextbigfuture.com is ranked #1 Science News Blog. It covers many disruptive technology and trends including Space, Robotics, Artificial Intelligence, Medicine, Anti-aging Biotechnology, and Nanotechnology.
Known for identifying cutting edge technologies, he is currently a Co-Founder of a startup and fundraiser for high potential early-stage companies. He is the Head of Research for Allocations for deep technology investments and an Angel Investor at Space Angels.
A frequent speaker at corporations, he has been a TEDx speaker, a Singularity University speaker and guest at numerous interviews for radio and podcasts. He is open to public speaking and advising engagements.