Ion trap technology are a scalable option for quantum computers.
Trapped atomic ions are a promising architecture that satisfies many of the critical requirements for constructing a quantum computer. At the heart of quantum computers are qubits, systems maintained in two or more quantum states simultaneously. Here, the qubits are manifested in the internal energy levels of the ions, and are manipulated through laser and microwave radiation. These technologies are a key factor in the success of atomic ions: scientists can set the frequency of the radiation to match that of the ion’s energy level spacings with extreme precision.
The qubits have long coherence time — meaning they can be placed in quantum states and remain that way long enough to perform calculations. The qubit’s states are not sensitive to ambient disturbances like magnetic fields, giving them inherent protection from the destructive environment.
Monroe and Kim are part of a larger collaboration called MUSIQC, which stands for Modular Universal Scalable Ion-trap Quantum Computer, and is supported by the Intelligence Advance Research Projects Activity (IARPA).
Surface trap fabricated by Sandia National Labs, supported by IARPA. This type of trap has been used to capture ions at JQI and Duke University, as well as other institutions. The image shown here appears on the cover of this week’s issue of Science Magazine. Credit and permissions, contact JQI
Science – Scaling the Ion Trap Quantum Processor
Trapped atomic ions are standards for quantum information processing, serving as quantum memories, hosts of quantum gates in quantum computers and simulators, and nodes of quantum communication networks. Quantum bits based on trapped ions enjoy a rare combination of attributes: They have exquisite coherence properties, they can be prepared and measured with nearly 100% efficiency, and they are readily entangled with each other through the Coulomb interaction or remote photonic interconnects. The outstanding challenge is the scaling of trapped ions to hundreds or thousands of qubits and beyond, at which scale quantum processors can outperform their classical counterparts in certain applications. We review the latest progress and prospects in that effort, with the promise of advanced architectures and new technologies, such as microfabricated ion traps and integrated photonics.
Science – The Future of Quantum Information Processing
Four Reviews look into the future of Quantum Information Processing (QIP) in some of its most promising physical realizations. Monroe and Kim discuss the challenges of scaling trapped ion architectures to hundreds and thousands of qubits and beyond. Devoret and Schoelkopf speculate on the future of superconducting circuits, whereas Awschalom et al. focus on the many promising qubit flavors based on spins in semiconductors. Finally, Stern and Lindner lay out the prospects for quantum computation using the entirely different approach of topologically protected states.
Superconducting Circuits for Quantum Information: An Outlook
The performance of superconducting qubits has improved by several orders of magnitude in the past decade. These circuits benefit from the robustness of superconductivity and the Josephson effect, and at present they have not encountered any hard physical limits. However, building an error-corrected information processor with many such qubits will require solving specific architecture problems that constitute a new field of research. For the first time, physicists will have to master quantum error correction to design and operate complex active systems that are dissipative in nature, yet remain coherent indefinitely. We offer a view on some directions for the field and speculate on its future.
Science – Quantum Spintronics: Engineering and Manipulating Atom-Like Spins in Semiconductors
The past decade has seen remarkable progress in isolating and controlling quantum coherence using charges and spins in semiconductors. Quantum control has been established at room temperature, and electron spin coherence times now exceed several seconds, a nine–order-of-magnitude increase in coherence compared with the first semiconductor qubits. These coherence times rival those traditionally found only in atomic systems, ushering in a new era of ultracoherent spintronics. We review recent advances in quantum measurements, coherent control, and the generation of entangled states and describe some of the challenges that remain for processing quantum information with spins in semiconductors.
Topological Quantum Computation—From Basic Concepts to First Experiments
Quantum computation requires controlled engineering of quantum states to perform tasks that go beyond those possible with classical computers. Topological quantum computation aims to achieve this goal by using non-Abelian quantum phases of matter. Such phases allow for quantum information to be stored and manipulated in a nonlocal manner, which protects it from imperfections in the implemented protocols and from interactions with the environment. Recently, substantial progress in this field has been made on both theoretical and experimental fronts. We review the basic concepts of non-Abelian phases and their topologically protected use in quantum information processing tasks. We discuss different possible realizations of these concepts in experimentally available solid-state systems, including systems hosting Majorana fermions, their recently proposed fractional counterparts, and non-Abelian quantum Hall states.
If you liked this article, please give it a quick review on ycombinator or StumbleUpon. Thanks
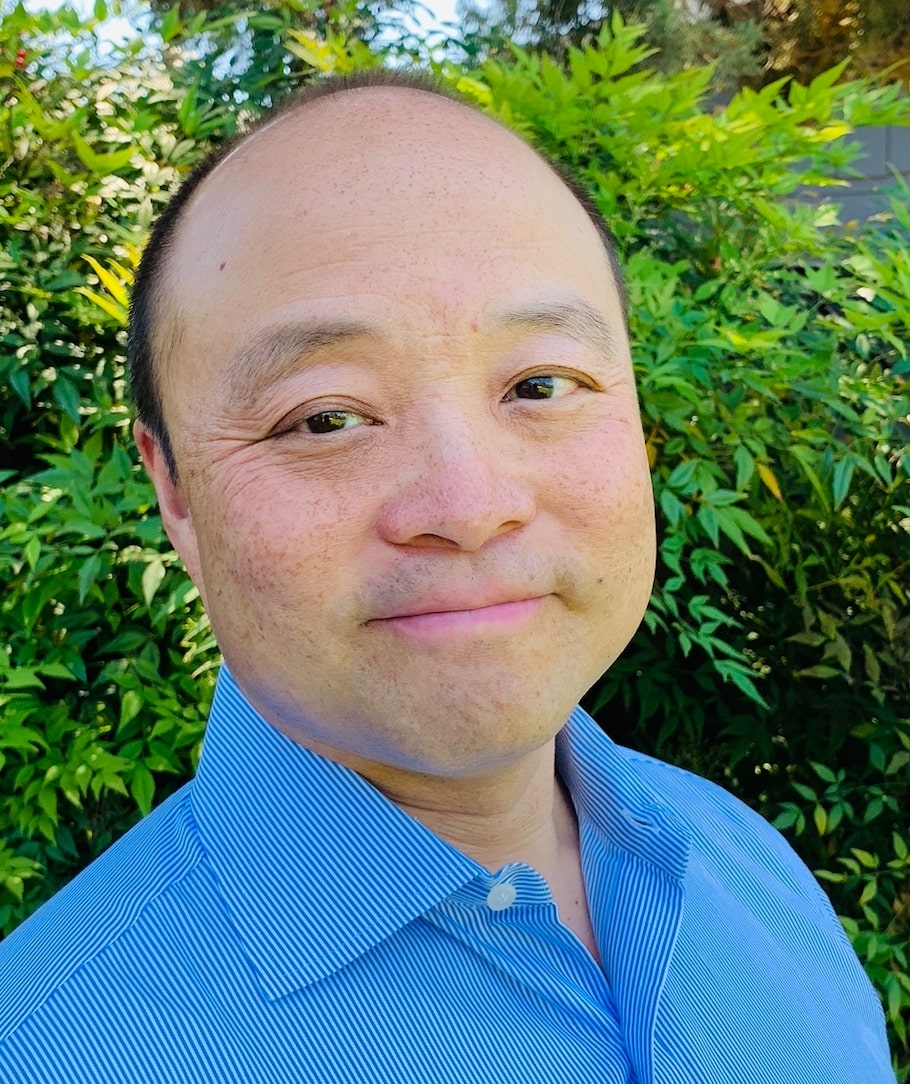
Brian Wang is a Futurist Thought Leader and a popular Science blogger with 1 million readers per month. His blog Nextbigfuture.com is ranked #1 Science News Blog. It covers many disruptive technology and trends including Space, Robotics, Artificial Intelligence, Medicine, Anti-aging Biotechnology, and Nanotechnology.
Known for identifying cutting edge technologies, he is currently a Co-Founder of a startup and fundraiser for high potential early-stage companies. He is the Head of Research for Allocations for deep technology investments and an Angel Investor at Space Angels.
A frequent speaker at corporations, he has been a TEDx speaker, a Singularity University speaker and guest at numerous interviews for radio and podcasts. He is open to public speaking and advising engagements.