The stability of a light sail riding on a laser beam is analyzed both analytically and numerically. Conical sails on Gaussian beams, which have been studied in the past, are shown to be unstable in general. A new architecture for a passively stable sail and beam configuration is proposed. The novel spherical shell sail design is capable of “beam riding” without the need for active feedback control. Full three-dimensional ray-tracing simulations are performed to verify our analytical results.
This is a passively stable laser-sail architecture that is capable of beam riding without active feedback control. The proposed design makes use of a spherical shell sail and a multimodal beam profile. While we have focused on a particular beam profile composed of a sum of four Gaussians, many others are possible, including sums of three or more Gaussians and radially symmetric ring-like profiles.
There are several effects which were not accounted for in this study, but which are likely to be important in the practical implementation of a laser-sail system. Perhaps most importantly, we have assumed a perfectly rigid sail. In practice, the sail will have flexible structural modes which may impact its beam-riding dynamics. Deformation of the sphere’s surface could cause non-zero torques on the sail, but could also result in favorable energy damping, which would reduce the amplitude of oscillations about the center of the beam. It may also be possible to pressurize the interior of the sphere with a gas or actively control the structure with actuators to alter both its stiffness and damping properties.
The Breakthrough Starshot Initiative was funded with $100 million to develop a laser sail system and a gram-scale spacecraft. It will try to get to a few percent of the speed of light using a high-power laser, with the goal of reaching the nearest stars within several decades
Zac is working on a Kicksat project with 100 sprite satellites
Zac has a blog that discusses his various articles, papers and projects
Zac has a 3U cubesat called Kicksat. On board are 100 circuit boards measuring 3.5cm on a side and weighing four grams, each holding sensors, a processor, solar cells, a radio and a pair of coiled 10cm whiskers. Once in orbit each of those circuit boards—he calls them “sprites”—would have headed off on its own, kicked out of the tiny mother ship by the uncoiling of its antennae, and the project’s backers on Kickstarter would have had their own personal Sputniks, transmitting at a frequency they could monitor. A 3mm smartphone camera would be an easy addition
Lubin’s designs would enable wafersats to reach 25% of lightspeed and a 100 ton spaceship to reach 1000 kilometers per second.
Nextbigfuture notes that for manned missions going beyond 1000 km per second, the wafer chips could be accelerated at a manned ship with a pusher plate (like the Project Orion ship) but the energy would be kinetic and not nuclear.
For large object construction, we need to develop the Tether Unlimited Spiderfab technology. This is construction in space with robots which means systems can be lighter and bigger like robots assembling an outdoor tent of sticks in space instead of building something on the ground and making it tough enough to withstand 3Gs or more of acceleration at launch.
They propose a roadmap to a program that will lead to sending relativistic probes to the nearest stars and will open up a vast array of possibilities of flight both within our solar system and far beyond. Spacecraft from gram level complete spacecraft on a wafer (“wafersats”) that reach more than ¼ c and reach the nearest star in 20 years to spacecraft with masses more than 100,000 kg (100 tons) that can reach speeds of greater than 1000 km/s. These systems can be propelled to speeds currently unimaginable with existing propulsion technologies. To do so requires a fundamental change in our thinking of both propulsion and in many cases what a spacecraft is. In addition to larger spacecraft, some capable of transporting humans, we consider functional spacecraft on a wafer, including integrated optical communications, imaging systems, photon thrusters, power and sensors combined with directed energy propulsion. The costs can be amortized over a very large number of missions beyond relativistic spacecraft as such planetary defense, beamed energy for distant spacecraft, sending power back to Earth, stand-off composition analysis of solar system targets, long range laser communications, SETI searches and even terraforming. The human factor of exploring the nearest stars and exo-planets would be a profound voyage for humanity, one whose non-scientific implications would be enormous. It is time to begin this inevitable journey far beyond our home.
Photon propulsion is an old idea going back many years, with some poetic references several hundred years ago. A decade ago what they now propose would have been pure fantasy. It is no longer fantasy. Recent dramatic and poorly-appreciated technological advancements in directed energy have made what we propose possible, though difficult. There has been a game change in directed energy technology whose consequences are profound for many applications including photon driven propulsion. This allows for a completely modular and scalable technology with radical consequences
The photon driver is a laser phased array which eliminates the need to develop one extremely large laser and replaces it with a large number of modest (kW class) laser amplifiers that are inherently phase locked as they are fed by a common seed laser. This approach also eliminates the conventional optics and replaces it with a phased array of small optics that are thin film optical elements. Both of these are a follow on DARPA and DoD programs and hence there is enormous leverage in this system. The laser array has been described in a series of papers we have published and is called DE-STAR (Directed Energy System for Targeting of Asteroids and ExploRation). Powered by the solar PV array the same size as the 2D modular array of modest and currently existing kilowatt class Yb fiber-fed lasers and phased-array optics it would be capable of delivering sufficient power to propel a small scale probe combined with a modest (meter class) laser sail to reach speeds that are relativistic. DE-STAR units are denoted by numbers referring to the log of the array size in meters (assumed square). Thus DE-STAR-1 is 10 meters on a side, -2 is 100 meters, etc. Photon recycling (multiple bounces) to increase the thrust is conceivable and has been tested in our lab but it NOT assumed. The modular sub systems (baselined here at 1-4 meters in diameter) fit into current rocket launchers
This technology is scalable over an enormous range of mass scales. The “laser photon driver” can propel virtually any mass system with the final speed only dependent on the scale of the driver built. Accelerating small 10µm “grains” to Mach 100-1000 for hypersonic tests would be of great interest, for example. Once built the system can be amortized over a very large range of missions allowing literally hundreds of relativistic wafer scale payload launches per day (~40,000/yr or one per sq deg on the sky) or 100-10,000 kg payloads for interplanetary missions at much slower rates (few days to weeks). For reference 40,000 wafers/ and reflectors, enough to send one per square degree on the entire sky, have a total mass of only 80kg
For photon engines (emission – no reflection) ISP = 30,000,000 while for the reflection case it is twice that or 60,000,000. In the idealized case of laser driven ablation engine we get the thrust ratio, compared to the photon reflection case, of c/ vrel ~ 15000 to 30000 consistent with our detailed ablation simulations. It is also possible to use laser heated H2 via heat exchangers to get even high ISP, due largely to the lower molecular mass, and thus higher exhaust speed for a given temperature
Laser Amplifier improvement is the improvement of the core enabling technology
Phase lockable lasers and current PV performance
New fiber-fed lasers at 1 micron have efficiencies near 40% (DARPA Excalibur program). They assume incremental efficiency increases to 70% though current efficiencies are already good enough to start the program. It is conceivable that power density could increase to 10 kW/kg in a decade given the current pace. Current space multijunction PV has an efficiency of nearing 40% with deployable mass per power of less than 7 kg/kW (ATK Megaflex as baselined for DE-STARLITE). Multi junction devices with efficiency in excess of 50% are on the horizon with current laboratory work exploring PV at efficiencies up to 70% over the next decade. They anticipate over a 20 year period PV efficiency will rise significantly, though it is NOT necessary for the roadmap to proceed.
Technology Maturation:
Laser and Phased Array
- Increase TRL of laser amplifiers to at least TRL 6
- Test of low mass thin film optics as an option
- Reduce SBS effect to lower bandwidth and increase coherence time/ length
- Optimize multiple lower power amplifiers vs fewer higher power units – SBS/coherence trades
- Maturation and miniaturization of phase control elements for phased array
- Phase tapping and feedback on structure
- Structural metrology designs
- Study of optimized Kalman filters as part of phase control and servo targeting loop
- Study and test near field phase feedback from small free-flyer elements
- Study beam profiling and methods to smooth beam on reflector
- Beam randomization techniques to flatten beam
Reflector
- Study multilayer dielectric coating to minimize loss and maximize reflectivity – trade study
- Study materials designs for minimal mass reflectors – plastics vs glasses
- Shape designs for reflector stability – shaping
- Study designs with varying thicknesses and dielectric layers
- Study designs with low laser line absorption and high thermal IR absorption (emission)
- Study broader band reflectors to deal with relativistic wavelength shift with speed
- Study self stabilizing designs
- Simulations of reflector stability and oscillations during acceleration phase – shape changes
- Study spinning reflector to aid stability and randomization of differential force and heating
- Study techniques for reflector to laser active feedback
- Study techniques to keep beam on reflector using “beacons”.
Wafer scale spacecraft
- Study materials for lowest power and high radiation resistance and compatibility with sensors
- Determine power requirements
- Study onboard power options – RTG, beta converter, beamed power
- Design narrow bandgap PV for beamed power phase
- Design on-wafer laser communications
- Design optical and IR imaging sensors
- Star tracker and laser lock modes
- Study swarm modes including intercommunications
- Design watchdog timers and redundant computational and sensor/ power topologies
- Test in beam line to simulate radiation exposure
- Study high speed dust impacts on the wafer and design in fault tolerance
- Design on-board or thin film “pop up” optics
- Design fiber optic or similar cloaking to mitigate heating during laser exposure
- Simulate thermal management both during laser exposure and during cruise phase
- Simulate radiation exposure during cruise phase
- Study materials for lowest power and high radiation resistance and compatibility with sensors
- Simulate imaging of target objects
- Study use of WaferSat for planetary and terrestrial probes
Communications
- Optimize wafer only laser communications
- Study feasibility of using acceleration reflector as part of laser communications
- Study feasibility of using reflector as thin film optics for laser comm and imaging
System Level
- Detailed design studies including mass tradeoffs and costing vs system size
- Develop cost roadmaps identifying critical elements as impediments to deployment vs size
- Design, build, test ground based structures with metrology feedback system
- Design and simulate orbital structures of various sizes (fixed vs sub element free flyer)
- Study orbital tradeoffs and project launcher feasibility vs time
- Study LEO, GEO, Lagrange points, lunar options
- Simulations of prober orbital trajectories including any Earth blockage effects
- Work with space PV designers to optimize efficiency and minimize mass
- Develop PV roadmap for mass, efficiency, rad resistance and aging
- Develop roadmap to reducing radiator mass by 10x as goal.
- Study target selection of possible exoplanet systems
- Study solar system targets
- Study multi mode use including space debris, beamed power, SPS, planetary defense …
- Begin discussion of geopolitical concerns such a system make invoke
SOURCES – A Roadmap to Interstellar Flight by Philip Lubin, Zac Machester and Abraham Loeb paper and site, Arxiv
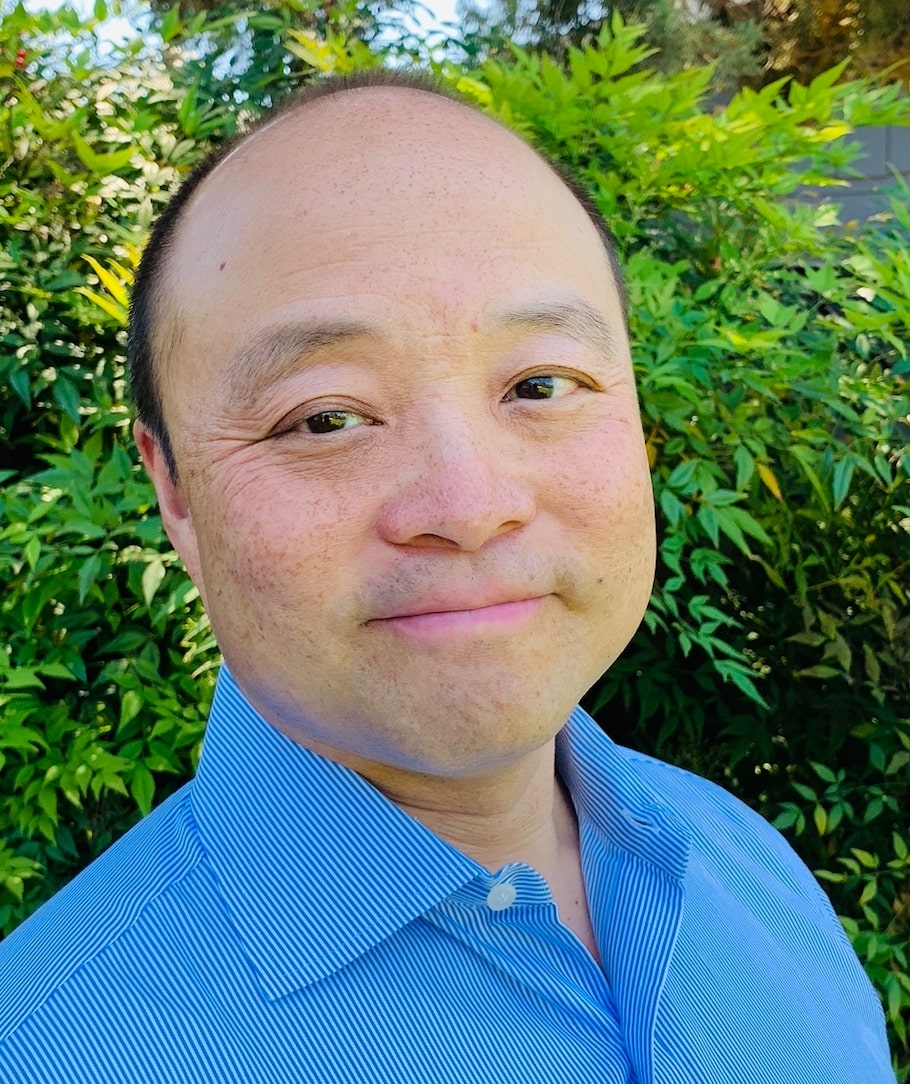
Brian Wang is a Futurist Thought Leader and a popular Science blogger with 1 million readers per month. His blog Nextbigfuture.com is ranked #1 Science News Blog. It covers many disruptive technology and trends including Space, Robotics, Artificial Intelligence, Medicine, Anti-aging Biotechnology, and Nanotechnology.
Known for identifying cutting edge technologies, he is currently a Co-Founder of a startup and fundraiser for high potential early-stage companies. He is the Head of Research for Allocations for deep technology investments and an Angel Investor at Space Angels.
A frequent speaker at corporations, he has been a TEDx speaker, a Singularity University speaker and guest at numerous interviews for radio and podcasts. He is open to public speaking and advising engagements.