Transition metal dichalcogenides (TMDs), materials offer several key features not available in graphene and are emerging as next-generation semiconductors. TMDs could realize topological superconductivity and thus provide a platform for quantum computing.
“Our proposal is very realistic – that’s why it’s exciting,” Kim said of her group’s research. “We have a theoretical strategy to materialize a topological superconductor … and that will be a step toward building a quantum computer.
The Cornell group’s proposal: The TMDs’ unusual properties favor two topological superconducting states, which, if experimentally confirmed, will open up possibilities for manipulating topological superconductors at temperatures near absolute zero.
Kim identified hole-doped (positive charge-enhanced) single-layer TMDs as a promising candidate for topological superconductivity, based on the known special locking between spin state and kinetic energy of electrons (spin-valley locking) of single-layer TMDs, as well as the recent observations of superconductivity in electron-doped (negative charge-enhanced) single-layer TMDs.
The group’s goal is a superconductor that operates at around 1 Kelvin (approximately minus 457 degrees Fahrenheit), that could be cooled with liquid helium sufficiently to maintain quantum computing potential in a superconducting state.
Theoretically, housing a quantum computer powerful enough to justify the power needed to keep the superconductor at 1 Kelvin is not out of the question, Kim said. In fact, IBM already has a 7-qubit (quantum bit) computer, which operates at less than 1 Kelvin, available to the public through its IBM Quantum Experience.
A quantum computer with approximately six times more qubits would fundamentally change computing, Kim said.
“If you get to 40 qubits, that computing power will exceed any classical computers out there,” she said. “And to house a 40-qubit [quantum computer] in cryogenic temperature is not that big a deal. It will be a revolution.”
Microsoft has been developing topological quantum computing for more than a decade and today has researchers working with academic laboratories to craft devices and writing software for future machines. Quantum-computing dignitaries, such as Alexei Kitaev, Daniel Gottesman and, most notably, Michael Freedman [the Fields Medal-winning director of Microsoft’s Station Q research lab], spearheaded the growth of our quantum-computing groups. Microsoft followed Freedman’s own trailblazing vision about how to do things that we followed.
Arxiv – Topological superconductivity in monolayer transition metal dichalcogenides
Theoretically it has been known that breaking spin-degeneracy and effectively realizing ‘spinless fermions’ is a promising path to topological superconductors. Yet, topological superconductors are rare to date. Here, we propose to realize spinless fermions by splitting the spin-degeneracy in momentum space. Specifically, we identify monolayer hole-doped transition metal dichalcogenide (TMD)s as candidates for topological superconductors out of such momentum-space-split spinless fermions. Although electron-doped TMDs have recently been found superconducting, the observed superconductivity is unlikely topological due to the near spin-degeneracy. Meanwhile, hole-doped TMDs with momentum-space-split spinless fermions remain unexplored. Employing a renormalization group analysis, we propose that the unusual spin-valley locking in hole-doped TMDs together with repulsive interactions selectively favors two topological superconducting states: inter-pocket paired state with Chern number 2 and intra-pocket paired state with finite pair-momentum. A confirmation of our predictions will open up possibilities for manipulating topological superconductors on the device friendly platform of monolayer TMDs.
Nature Communications – Topological superconductivity in monolayer transition metal dichalcogenides
The quest for material realizations of topological chiral superconductors with nontrivial Chern numbers is fuelled by predictions of exotic signatures, such as Majorana zero modes and quantized Hall effects. Unfortunately, natural occurrence of bulk topological superconductors are rare with the best candidates being superfluid 3He and Sr2RuO4. Instead, much recent experimental progress relied on proximity-inducing pairing to a spin–orbit-coupled band structure building on the proposal of Fu and Kane. Their key insight was that a paired state of spinless fermions is bound to be topological and that the surface states of topological insulators are spinless in that the spin degeneracy is split in position space (r-space): the two degenerate Dirac surface states with opposite spin textures are spatially separated. Nevertheless, despite much experimental progress along this direction the confinement of the helical paired state to the interface of the topological insulator and a superconductor limits experimental access to its potentially exotic properties.
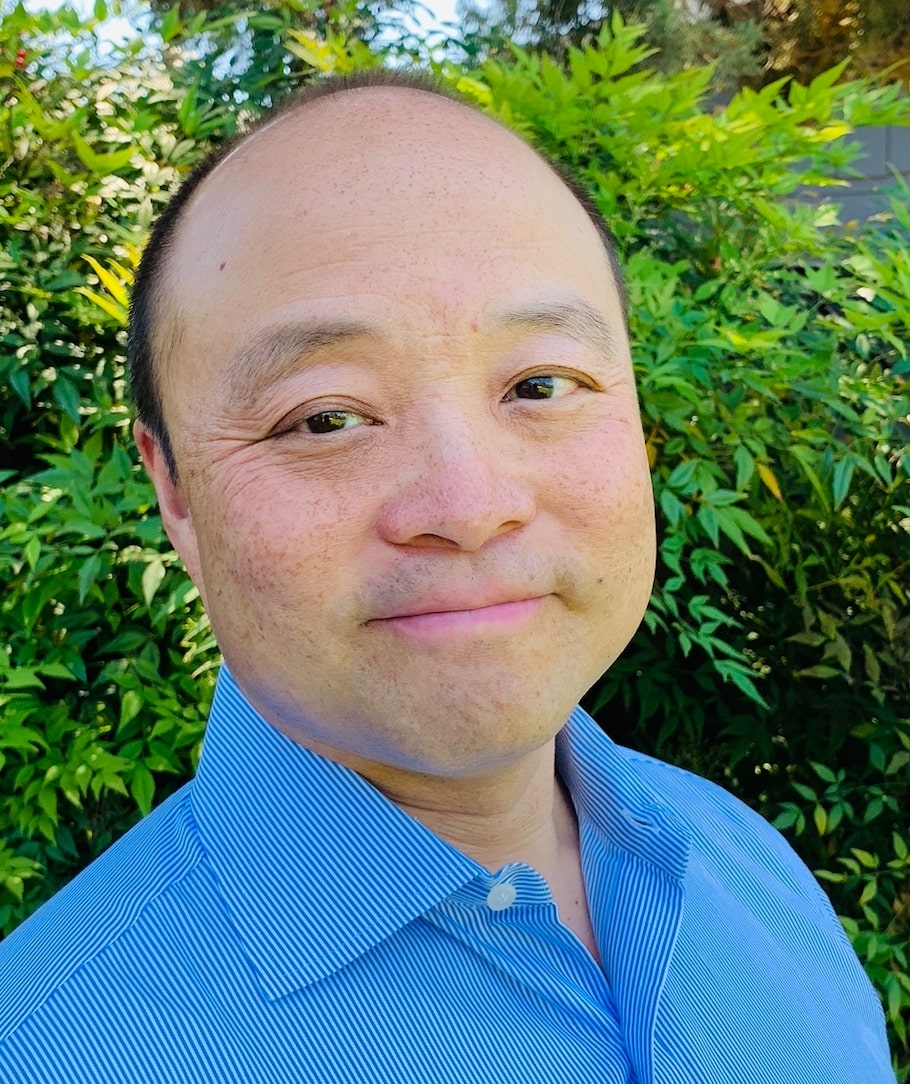
Brian Wang is a Futurist Thought Leader and a popular Science blogger with 1 million readers per month. His blog Nextbigfuture.com is ranked #1 Science News Blog. It covers many disruptive technology and trends including Space, Robotics, Artificial Intelligence, Medicine, Anti-aging Biotechnology, and Nanotechnology.
Known for identifying cutting edge technologies, he is currently a Co-Founder of a startup and fundraiser for high potential early-stage companies. He is the Head of Research for Allocations for deep technology investments and an Angel Investor at Space Angels.
A frequent speaker at corporations, he has been a TEDx speaker, a Singularity University speaker and guest at numerous interviews for radio and podcasts. He is open to public speaking and advising engagements.