Every doubling of current means 30 times power. So some relatively simple modifications which are being developed should boost power levels by 300 times.
Total elimination of arcing, which they are working on now, may drop the resistance to as low as 2 mOhms.
Full power, shorter electrodes, and our existing switches, FF-1 will produce over 2.3 MA, over twice our current output.
New, faster switches now under design for LPP by Raytheon will get us the rest of the way to our goal of 2.8 MA.
In May, 2012, the LPP plan for the next 12 months was laid out.
To gain higher yield and to attain “feasibility” the following steps are being done over the course of the next year (2012):
1) The “teeth that chew the sheath” tungsten crown to regularize the filaments – 10-100x yield
2) Full power output of Capacitors and to ‘Imitate’ the heavier mixture of pB11 by using Deuterium/Nitrogen.
3) Shorter Electrodes, slower run down, more fill gas.
4) New Raytheon switches for more Current from capacitors – 10x yield.
5) Switch to pB11 (incrementally higher percentage from the D/N mix) – 15x yield.
Goal: 30 kJ* gross fusion energy per shot proves feasibility of a positive net power output Generator using aneutronic fuel!
*A 5MW production reactor would have about 66 kJ gross fusion yield per shot*
Improved Theories
Since they first observed the 160 keV energies of the ions (equivalent to 1.8 billion C) over a year ago, we had been puzzled as to why they were so much higher than the 40 keV we had predicted.
The first big step to the solution came May 15, with the publication online in the Journal of Fusion Energy of a paper by the Iranian team, S. Abolhasani, M. Habibi, and R. Amrollahi, “Analytical Study of Quantum Magnetic and Ion Viscous Effects on p11B Fusion in Plasma Focus Devices.” The paper studied in greater detail the quantum magnetic field effect originally applied to the DPF by Lerner, for the first time independently confirming our calculations showing that ignition and net energy gain can be achieved with pB11 (hydrogen-boron) fuel, the key to obtaining aneutronic fusion energy.
But in addition, the paper applied to the plasma focus device a process studied by British physicist Malcolm Haines to explain high ion energies achieved in the Z-machine. That process, called “ion viscous heating” works like this: as the plasmoid contracts, ions moving inward at different velocities start to mix together, so that their ordered velocity of motion is converted into the random velocity of heat. By analogy this is a bit like trying to rapidly stir a vicious liquid like honey. The resistance of the liquid to rapid changes in velocity—its viscosity—converts kinetic motion to heat and the liquid warms up. The formulae derived in the paper indicated that this viscous heating could possibly explain FF-1’s high temperatures.
But there was a second puzzle to be solved. The viscous process heats only the ions—the heavy nuclei—not the electrons. If the electrons are too cold, collisions between them and the ions would rapidly cool the ions. So what heated the electrons up hot enough so they would not cool those ions too fast?
On June 10, Lerner thought of a possible solution. The electron beam will induce currents in the plasmoid electrons, just as any rapidly changing current induces other currents in a surrounding conductor (we intend to use this same process to capture the energy of the ion beam with a coil of wire). But since the plasmoid has a much greater density of electrons that the beam, the same current will be distributed over more electrons, and they will be moving much slower than the beam electrons. These slower electrons will have the time to undergo collisions and convert their kinetic energy to heat. Following up on this hypothesis, Talaei found a dozen important papers on this same process of electron beams heating plasma by induced currents, although none applied directly to the plasma focus. Curiously, all the papers dated from the 1970’s, the same fertile period that gave rise to the first research on the magnetic field effect.
When we combined the formulae from these papers for electron temperatures with the formulae from the viscous heating paper and plugged in the observed values for plasma density, radius and current from FF-1’s experiments, the predicted ion energy came out to 170 keV—in terrific agreement with our best observed results of 160 keV. Of course more experiments will be needed to fully confirm that this theoretical explanation is right, but this combination of processes is clearly a possible explanation.
Interestingly, the effectiveness of the ion viscous heating declines rapidly with increasing density of the plasmoid and smaller plasmoid size, while the effectiveness of the induced current heating rises for smaller, denser, plasmoids. So as we increase plasmoid density we expect to see a temporary decline in temperature, and then a subsequent rise back to the levels needed to burn pB11. Fusion yield will continue to rise, as the higher density and thus higher burn rate will more than compensate for the temporary decline in T.
If you liked this article, please give it a quick review on ycombinator or StumbleUpon. Thanks
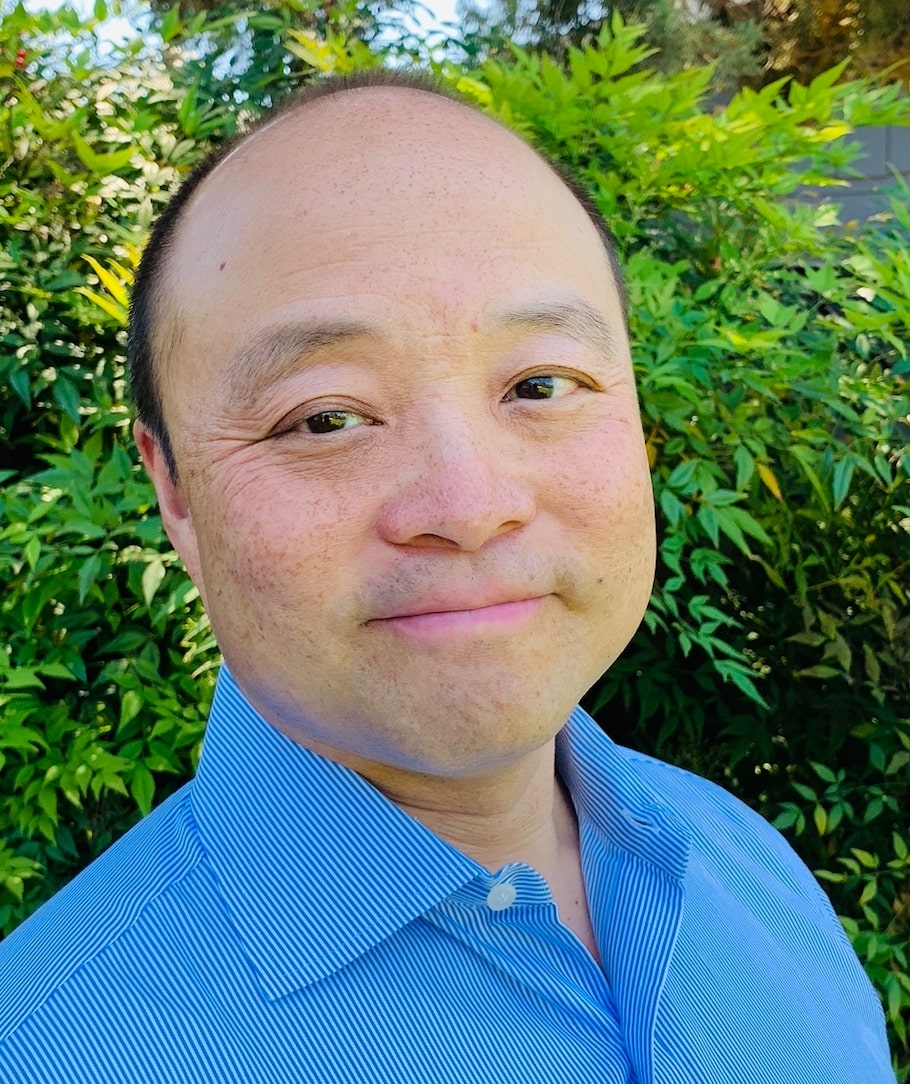
Brian Wang is a Futurist Thought Leader and a popular Science blogger with 1 million readers per month. His blog Nextbigfuture.com is ranked #1 Science News Blog. It covers many disruptive technology and trends including Space, Robotics, Artificial Intelligence, Medicine, Anti-aging Biotechnology, and Nanotechnology.
Known for identifying cutting edge technologies, he is currently a Co-Founder of a startup and fundraiser for high potential early-stage companies. He is the Head of Research for Allocations for deep technology investments and an Angel Investor at Space Angels.
A frequent speaker at corporations, he has been a TEDx speaker, a Singularity University speaker and guest at numerous interviews for radio and podcasts. He is open to public speaking and advising engagements.