Researchers demonstrate that superconductor critical temperature can be raised above room-temperature to ≈ 400K in cuprates by precise control of the spatial separation of dopants. Hence, there still remains substantial “latent” Tc in cuprates. Their proposed doping strategy and superconducting mechanism is not restricted to cuprates and may be exploited in other materials.
Their room-temperature Tc result is based upon four observations:
• Cuprates are intrinsically inhomogeneous on the atomic-scale and are comprised of insulating and metallic regions. The metallic region is formed by doping the material.
• A diverse set of normal state properties are explained solely from the topological properties of these two regions and their doping evolution.
• Superconductivity results from phonons at or adjacent to the interface between the metallic and insulating regions. Transition temperatures Tc ∼ 100 K are possible because the electron-phonon coupling is of longer-range than metals (nearest neighbor).
• These interface phonons explain the observed superconducting properties and lead to their prediction of room-temperature superconductivity.
Generally, it is most favorable to fabricate the narrowest wires that are spaced closely together because both critical temperature and critical current will be large. In addition, interfacial phonon modes will couple to both the closest wire and the next-nearest neighboring wire, leading to further increase in critical temperature.
Results lead to the following approaches for achieving higher Tc and Jc. Unless explicitly stated, the bullet points below apply to any type of material (cuprate or noncuprate).
• The material should be inhomogeneous with a metallic region and an insulating region. The insulating region does not have to be magnetic. However, we believe the antiferromagnetic insulating region helps maintain the sharp metal-insulator boundary seen in cuprates. An ordinary insulator or a semiconductor with a small number of mobile carriers is sufficient to obtain a longer ranged electron-phonon coupling at the interface because there is less electron screening in the semiconducting (or insulating) region compared to the metallic region.
• The ratio of the number of metallic unit cells on the interface (adjacent to at least one insulating unit cell) to the total number of metallic unit cells must be larger than 20%. We use the terms interface and surface interchangeably below.
The number of metallic unit cells on the interface (or surface) must be a large fraction of the total number of metallic unit cells in order for the enhanced electron-phonon pairing at the interface to have an appreciable affect on Tc. From our calculations in 50% of optimal Tc is obtained when the ratio is ≈ 50%, and 25% of optimal Tc occurs when the ratio is ≈ 35%. Below a surface metal unit cells to total metal unit cells ratio of 20%, Tc falls off exponentially, and therefore Tc is too low to be useful.
Metallic clusters that are smaller than approximately the coherence length do not contribute to Tc due to thermal fluctuations. The surface metal unit cells to total metal unit cells ratio above should only include surface metal unit cells in extended metallic clusters.
• Add dopants to an insulating parent compound that leads to metallic regions. Doping a metallic parent compound to create insulating regions will work also. In cuprates, the parent compound is insulating and doping creates metallic regions.
• Avoid small disconnected metallic clusters. If they are smaller than the coherence length, they do not contribute to Tc due to thermal fluctuations. In cuprates, high Tc can be obtained at very low doping if all the dopants leading to isolated plaquettes and small plaquette clusters are arranged such that a single contiguous metallic cluster is formed. While the Tc may be high, Jc will be low if the size of the metallic region is a small fraction of the total volume of the crystal.
• Superconducting wires lead to a small increase of Tc and a large increase of Jc. Metallic wires lead to a tiny increase in Tc, as discussed in the second bullet above. However, metallic wires increase Jc dramatically (up to a factor of ∼ 100) by eliminating the tortured conduction pathways. For cuprates, optimal Tc doping at x = 0.16 is barely above the 2D percolation threshold of x ≈ 0.15 doping.
“Crowded” crossed wires that have high Tc and Jc. The crossed metallic wires are one plaquette in width. Yellow metallic overlay. The schematic shows the fluctuating dumbbells as crosses and the metallic region in yellow.
Hence, the conducting pathways in a single CuO2 plane are tenuous at optimal doping. Current materials fabrication methods for cuprates have optimized the Tc at the expense of Jc. We find this point to be evidence that despite all the proposals in over 200,000 refereed publications, there has been little guidance to the materials synthesis community on what is relevant at the atomic level for optimizing Tc and Jc
Arxiv – Latent Room-Temperature Tc in Cuprate Superconductors
The ancient phrase, “All roads lead to Rome” applies to Chemistry and Physics. Both are highly evolved sciences, with their own history, traditions, language, and approaches to problems. Despite all these differences, these two roads generally lead to the same place. For high temperature cuprate superconductors however, the Chemistry and Physics roads do not meet or even come close to each other. In this paper, we analyze the physics and chemistry approaches to the doped electronic structure of cuprates and find the chemistry doped hole (out-of-the-CuO2-planes) leads to explanations of a vast array of normal state cuprate phenomenology using simple counting arguments. The chemistry picture suggests that phonons are responsible for superconductivity in cuprates. We identify the important phonon modes, and show that the observed Tc ∼ 100 K, the Tc-dome as a function of hole doping, the change in Tc as a function of the number of CuO2 layers per unit cell, the lack of an isotope effect at optimal Tc doping, and the D-wave symmetry of the superconducting Cooper pair wavefunction are all explained by the chemistry picture. Finally, we show that “crowding” the dopants in cuprates leads to a pair wavefunction with S-wave symmetry and Tc ≈ 280 − 390 K. Hence, we believe there is enormous “latent” Tc remaining in the cuprate class of superconductor
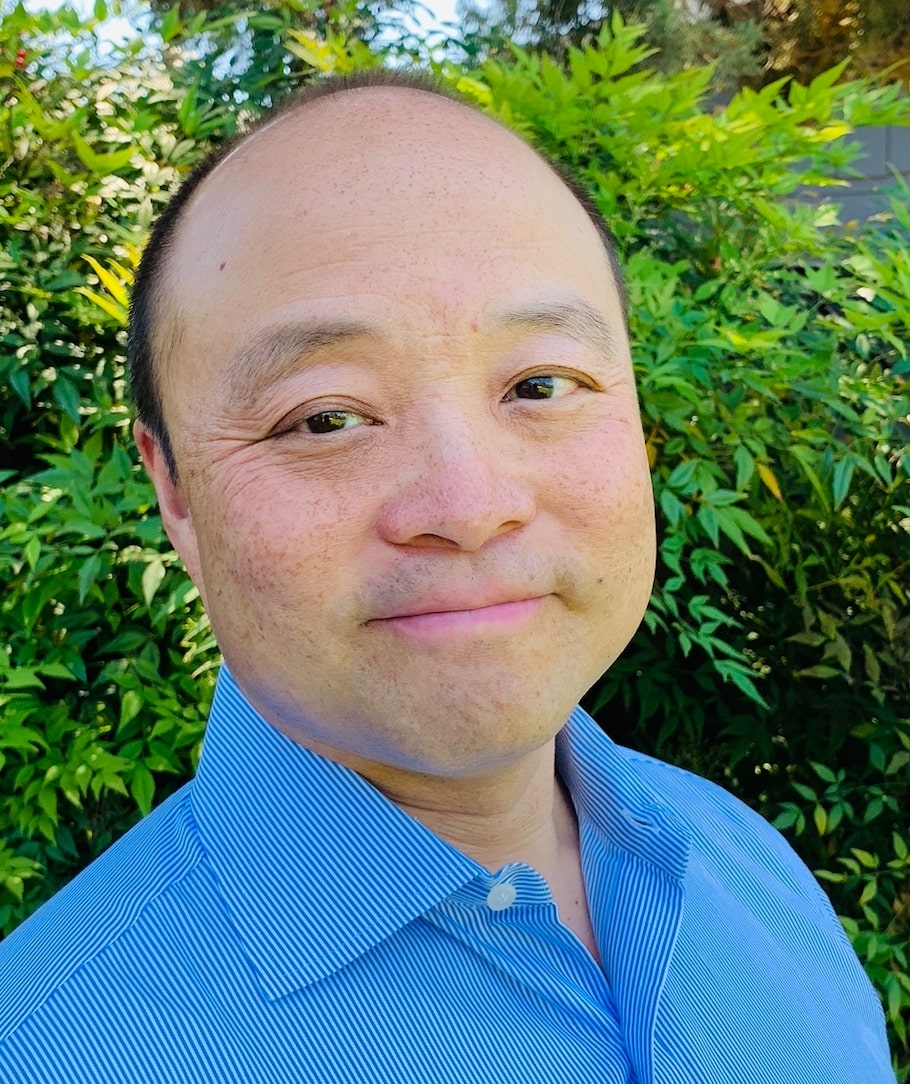
Brian Wang is a Futurist Thought Leader and a popular Science blogger with 1 million readers per month. His blog Nextbigfuture.com is ranked #1 Science News Blog. It covers many disruptive technology and trends including Space, Robotics, Artificial Intelligence, Medicine, Anti-aging Biotechnology, and Nanotechnology.
Known for identifying cutting edge technologies, he is currently a Co-Founder of a startup and fundraiser for high potential early-stage companies. He is the Head of Research for Allocations for deep technology investments and an Angel Investor at Space Angels.
A frequent speaker at corporations, he has been a TEDx speaker, a Singularity University speaker and guest at numerous interviews for radio and podcasts. He is open to public speaking and advising engagements.