Nuclear Fusion Journal – Creation of a high-temperature plasma through merging and compression of supersonic field reversed configuration plasmoids (H/T Talk Polywell)
A new device, the Inductive Plasma Accelerator, was employed to simultaneously form and accelerate two oppositely directed field reversed configurations (FRCs) where the relative velocity (600 km s−1) of the plasmoids was much larger than their internal thermal motion. Upon collision all of the FRC directional energy was observed to be rapidly thermalized concurrent with complete magnetic reconnection of the two FRCs. Upon merging, the resulting FRC was compressed to kilovolt ion temperatures exhibiting a configuration lifetime better than predicted by past scaling of in situ formed FRCs. With the improved FRC confinement scaling, a pulsed plasma device based on this approach capable of achieving fusion gain is examined. For an FRC with a poloidal flux 20 mWb or greater, the fusion energy yield per pulse exceeds the plasma energy for compression fields of 10 Telsa or more. The scaling is insensitive to the compression chamber radial scale, providing for the possibility of a very compact fusion neutron source.
Given the scaling and the desire to optimize at smaller radius and higher power
density, it is worth determining the parameters of a device capable of a gain of five. From equation 10 the following values will yield such a gain:length (s) 2 meters Poloidal Flux 25 mWb Compression fields 16 Telsa Q (gain) 5 times more energy out than put in .
Helion Energy has now performed what was previously described as the Phase 2 work The next step would be a full power component test facility which would cost about $20-30 million and could be done in 1-2 years.
Gain plot as in figure 7 with regions of exclusion for different compression coil radii rc, where an ion temperature was 9 keV and a FRC length ls = 1 m were assumed.
Midplane cross-section of compression section with fissile-fussile breeding blanket.
With the successful merging of supersonic FRCs in the IPA experiments, a method with the potential for the production of fusion energy in a simple and technologically appealing manner has been demonstrated. FRC plasmoids were brought to high temperature and density via a linear, sequenced array of low field compression coils establishing a means for obtaining fusion conditions remote from the plasma formation system. To obtain the requisite Lawson product a larger FRC must be formed, accelerated and compressed to higher fields. From the results of the
Supersonic Merging and Compression of FRCs IPA experiments together with equation 10, breakeven conditions will require an increase in poloidal flux which can be achieved by a radial scale increase of roughly a factor of three to a formation size similar to the LSX experiment. The merging chamber would remain at 0.1 meter radius but at much higher field (10 Tesla). The same magneto-kinetic compression scheme as used on IPA would be extended in order to impel the FRC into the higher fields of the burn chamber.The suitability of this approach to fusion or fusion-fission hybrid applications is the consequence of the numerous critical advantages that significantly reduce the technological challenges, as well as the cost of development and operation. The more significant begin with the ability of the FRC plasmoid to be translated and merged where the FRC formation and kinetic energy input is added outside the burn chamber and realized only within the burn chamber as demonstrated in the IPA experiments. The plasma divertor region is well removed from the reactor, and can be scaled to eliminate any power loading issues. With the diverter located remotely in an essentially neutron-free environment, tasks such as full tritium recovery and diverter maintenance are made much easier to perform.
In order to rapidly spawn additional fusion systems, maintaining a low tritium inventory and creating more tritium than consumed will be essential. Due to the pulsed FRC reactor’s linear, cylindrical geometry and the small scale of the fusion plasma compared to the neutron absorption region, the breeding blanket coverage can be optimized. It is critical that a sufficient number of energetic neutrons interact with lithium in the blanket to breed new tritium fuel. A high conversion efficiency of the fast fusion neutrons in the blanket surrounding the device makes significantly more fusion neutrons available for both fussile and fissile fuel generation as well as waste burning applications. The simply connected, linear geometry of the reactor vessel is also amenable to rapid and frequent first wall replacement if necessary. A fluid metal wall interface in this geometry is also feasible. This would allow for operation at the highest power density, and resolve several plasma-material wall issues.
There are also several critical attributes related to the unique nature of the FRC as a fusion plasma. Reactor costs will generally scale inversely with the fusion power density which in turn scales as 2B4. The FRC has the highest B of all magnetically confined fusion plasmas, and the simple cylindrical nature of the confining field coils provides for the highest magnetic fields. Utilizing the FRC plasmoid for magnetic fusion energy thus represents the highest fusion power density that can be attained in a non-destructive, repetitive manner. As indicated in figure 10, the small ratio of the FRC volume to the neutron absorbing blanket volume provides for the maximum fusion power density obtainable without exceeding thermal limitations in the blanket. For hybrid operation, the small footprint allows for easy integration into existing fission power plant infrastructure. The reduced reactor scale also means much faster and far less expensive iterations during the development phase which will be essential to the integration of improvements and new technologies.
At first thought, it would appear that a pulsed system would be inferior to a steady system, primarily due to the advantages of self-sustainment (i.e. ignition). There are several limitations imposed by a steady fusion burn, not the least being constrained to an inherently low power density. As is now being realized, the steady burn does not easily allow for maintenance of the plasma-wall interface. The ability to maintain the critical balance between alpha heating and plasma losses in an ignited reactor is an open question; as is tritium retention, fueling, burn diagnostics, plasma current sustainment, profile control, as well as several other device specific issues such as disruptions. By virtue of the cyclic nature of the burn, virtually all these issues are eliminated or significantly mitigated. Both fusion reactor gain and diverter wall loading can be readily regulated by the compression strength and rep rate Rp. All of the D-T fuel can be introduced during the initial formation of the FRC plasmoid, eliminating the need for refueling.
The major challenge for pulsed systems is operation at the highest efficiency possible in order to minimize what would otherwise be a large recirculating power fraction. Using pulsed magnetic fields to form, accelerate and compress the FRC to fusion conditions is efficient with Ohmic losses in the drive circuits as the primary loss channel. Employing a pulsed magnetic field also affords a unique opportunity for direct energy recovery. It has been appreciated in the past that pulsed systems employing flux compression can realize direct recovery of the fusion alpha energy. For the pulsed compression of the FRC this takes the form of the back emf experienced by the compression circuit due to the increase in FRC plasmoid pressure from the presence of the fusion alphas. This push-back during the natural oscillatory behavior of the compression circuit causes the energy storage capacitors to be recharged to greater energy from the increased expansion energy of the FRC. A key technology for such a system is the use of an opening switch to allow this energy to then be stored for the next pulse. This appears feasible as the voltages and energies envisioned for the prototype are within the range of what can be achieved with current solid state switches and power delivery systems. Additional recuperative operation of all the magnetic field circuits would provide for a way to significantly reduce the energy losses to primarily that of the FRC itself. Even that energy may be recoverable in some measure by direct conversion in the divertor as the plasma energy loss is primarily in the form of a high energy, directed plasma jet at the divertor entrance. The capability to make use of the plasma, fusion and electrical energy in an efficient manner is unique to the concept described here, and is critical for the commercial application of fusion to be realized without resorting to a larger scale, higher fusion gain system.
Possibly the most underappreciated advantage of a pulsed system comes from the ability to evaluate key performance milestones with single pulses. This minimizes neutron activation during development and further reduces cost as much simper and less robust pulse power systems can be employed.
Given the scaling and the desire to optimize at smaller radius and higher power
density, it is worth determining the parameters of a device capable of a gain of five. From equation 10 the following values will yield such a gain:length (s) 2 meters Poloidal Flux 25 mWb Compression fields 16 Telsa Q (gain) 5 times more energy out than put in .The fusion energy per pulse is given by equation 8 and for the assumed parameters yields Efus = 14 MJ. The rep rate Rp is determined by the wall loading limits.
Taking advantage of the translatability of the burning FRC, the compression section can be made much longer than the FRC so that the drifting FRC deposits the fusion energy along a series of compression coil sections. Alternatively, the FRCs can be merged and brought to rest in a different part of the elongated compression chamber with each pulse. In either case, the rep rate will likely never exceed 2 – 4 Hz for average power of 40 to 60 MW in order to maintain the average neutron fluence below 4 MW/m2. The low frequency minimizes the pulse power issues as well as any pump out concerns.
A low total fusion yield would at first seem restrictive. It does however have several advantages. With eventual fusion plant power output in the 200 to 500 MW range, the pulsed FRC reactor can be modularized. The investment-intensive energy input and fuel recovery systems can be used to power multiple, relatively inexpensive, easily replaceable burn chambers. This will be particularly important in the early stages of deployment when reliability and maintenance schedules will be less well understood.
In summary, with the repetitive and efficient generation of FRC plasmoids, brought to high temperature and density as they are injected into the burn chamber, a compact, low-cost fusion neutron source can be achieved. At a minimum it would be an attractive source for fast neutron based applications such as isotope production, fissile fuel breeding and fission waste transmutation. The expectation is that with the successful demonstration of a prototype scale device, a viable alternative for fusion energy production can be rapidly developed at an affordable cost, and will spur a renewed urgency to perform the required reactor development on a timescale that will allow fusion to be a major player in the world’s energy future.
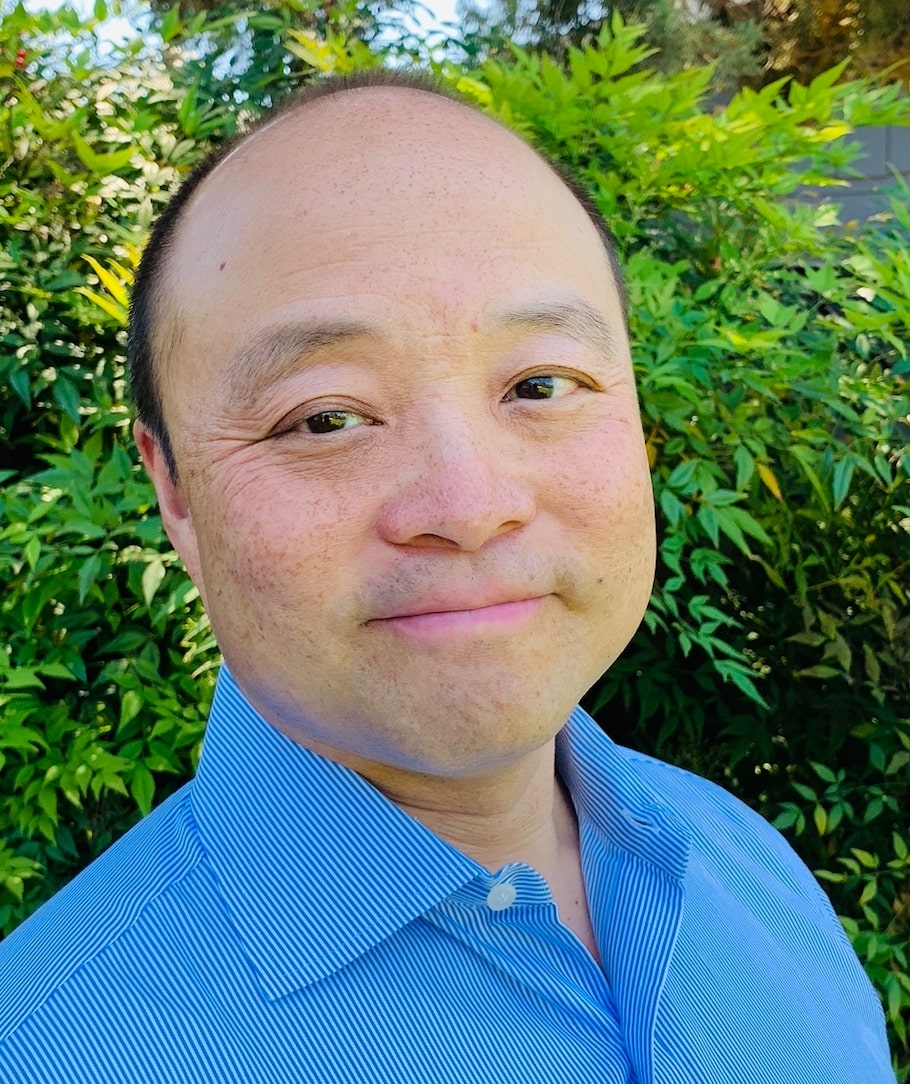
Brian Wang is a Futurist Thought Leader and a popular Science blogger with 1 million readers per month. His blog Nextbigfuture.com is ranked #1 Science News Blog. It covers many disruptive technology and trends including Space, Robotics, Artificial Intelligence, Medicine, Anti-aging Biotechnology, and Nanotechnology.
Known for identifying cutting edge technologies, he is currently a Co-Founder of a startup and fundraiser for high potential early-stage companies. He is the Head of Research for Allocations for deep technology investments and an Angel Investor at Space Angels.
A frequent speaker at corporations, he has been a TEDx speaker, a Singularity University speaker and guest at numerous interviews for radio and podcasts. He is open to public speaking and advising engagements.
Brian, you have me confused. This whole article is about Helion Energy and their reactor, right? I thought their deal was to burn D-He3, yet you talk about D-T. What’s up with that? Inquiring minds writing about fusion need to know!