Yoshiki Sasai has impressed many researchers with his green-fingered talent for coaxing neural stem cells to grow into elaborate structures. As well as the optic cup, he has cultivated the delicate tissue layers of the cerebral cortex and a rudimentary, hormone-making pituitary gland. He is now well on the way to growing a cerebellum — the brain structure that coordinates movement and balance. “These papers make for the most addictive series of stem-cell papers in recent years,” says Luc Leyns, a stem-cell scientist at the Free University of Brussels.
Sasai’s work is more than tissue engineering: it tackles questions that have puzzled developmental biologists for decades. How do the proliferating stem cells of an embryo organize themselves seamlessly into the complex structures of the body and brain? And is tissue formation driven by a genetic program intrinsic to cells, or shaped by external cues from neighbouring tissues? By combining intuition with patient trial and error, Sasai has found that it takes a delicate balance of both: he concocts controlled environments that feed cells physical and chemical signals, but also gives them free rein to ‘do their thing’ and organize themselves into issues. He sometimes refers to himself as a Japanese matchmaker who knows that, having been brought together, two strangers need to be left alone. “They know what to do,” he says. “They interact in a delicate manner, and if the external cues are too strong, it will override the internal ones.”
Sasai’s work could find medical applications. Recapitulating embryonic development in three dimensions, it turns out, generates clinically useful cells such as photoreceptors more abundantly and efficiently than two-dimensional culture can, and houses them in an architecture that mirrors that of the human body. Sasai and his collaborators are now racing to implant lab-grown retinas into mice, monkeys and humans. The way Sasai sees it, maturing stem cells in two-dimensional culture may lead to ‘next generation’ therapy — but his methods will lead to ‘next, next generation’ therapy.
Sasai and his colleagues discovered that the chordin protein is a key developmental signal released by the Spemann organizer. Rather than pushing nearby cells to become neurons, they found, chordin blocks signals that would turn them into other cell types. The work helped to establish the default model of neural induction: the idea that, without other signals, embryonic cells will follow an internal program to become neural cells.
By the late 1990s, embryonic-stem-cell scientists were also looking at these signals. They wanted to turn stem cells into mature cell types — particularly neurons — that might lead to therapies. The problem, says Sasai, is that scientists generally “push too hard and perturb the system”. Sasai knew that in the embryo, subtracting signals from the system is what counts, not perturbing it. “We tried to minimize external cues,” he says.
Sasai built an experimental system around that philosophy. He threw out the serum usually added to growing embryonic stem cells, which contains a brew of uncharacterized growth factors and other signalling molecules. He also removed physical cues, such as contact with the plastic surfaces of a culture dish, by allowing embryonic stem cells to spontaneously form floating aggregates known as embryoid bodies. “If they’re attached, they’re like prisoners and can’t act out their own desires,” he says. Keeping the cells alive without these support systems was a challenge but, five years of careful experimentation later, Sasai published and later patented his serum-free embryoid body culture — a pared-down life-support system with just the right mixture of ingredients for cells to survive. It would go on to form the heart of his brain-tissue factory.
…
All this will not create eyes that can be plugged into an eye socket like a light bulb into a lamp. Even if Sasai could get his optic cups to develop into mature retinas, researchers have little idea about how to wire a transplanted retina up to the brain.What the work does offer is a potentially abundant source of pure, dense, well-organized photoreceptors, the developmental stage of which can be precisely selected — something that has been difficult to achieve in standard two-dimensional culture. Eventually, Sasai hopes, his optic cups will provide sheets of photoreceptors that can be inserted into a retina damaged by conditions such as retinitis pigmentosa or macular degeneration. Sasai demonstrates the procedure by grabbing a stack of papers to stand in for the retinal layers and then slipping one sheet between the others.
Sasai has set his sights on more complex neural tissues. Last November, he reported the formation of a part of the pituitary gland — his “most complicated” tissue yet. In the embryo, the pituitary gland arises when two different tissues integrate to form a pouchlike structure. Sasai managed to recapitulate this in vitro partly by starting out with more than three times more embryonic stem cells than he had used to grow a mouse retina; the adjustment seems to increase the levels of signals that the cells exchange. When transplanted into mice in which the pituitary glands had been knocked out, the rudimentary organs restored the endocrine system and saved the mice. This work, too, might eventually provide a supply of pure, specialized cells, which could be used to treat endocrine disorders.
Sasai hopes to improve on his early efforts by growing a better pituitary gland, equipped with a blood supply; a cerebral cortex with all six layers of tissue; and photoreceptors mature enough to detect light. But his next major task is to culture a cerebellum, which will involve growing and integrating three tissues of different embryonic origins. The matchmaker is already at work, trying to conjure up the right atmosphere. “When a boy meets a girl, they start their own story — but not in a large auditorium full of people,” he says. “You need to put them on a beach or in a disco. Our system is simply going to create this environment.”
What Sasai plans to take on after the cerebellum is a secret, but he hopes eventually to encompass the whole brain. He does not mean building one — that would be enormously difficult and ethically fraught. Instead, he wants to work out how brain parts, with their remarkable capacity for autonomous growth and organization, combine and fold into a structure of such tremendous complexity.
Cerebral Cortex Engineering Paper
ABSTRACT – Here, we demonstrate self-organized formation of apico-basally polarized cortical tissues from ESCs using an efficient three-dimensional aggregation culture (SFEBq culture). The generated cortical neurons are functional, transplantable, and capable of forming proper long-range connections in vivo and in vitro. The regional identity of the generated pallial tissues can be selectively controlled (into olfactory bulb, rostral and caudal cortices, hem, and choroid plexus) by secreted patterning factors such as Fgf, Wnt, and BMP. In addition, the in vivo-mimicking birth order of distinct cortical neurons permits the selective generation of particular layer-specific neurons by timed induction of cell-cycle exit. Importantly, cortical tissues generated from mouse and human ESCs form a self-organized structure that includes four distinct zones (ventricular, early and late cortical-plate, and Cajal-Retzius cell zones) along the apico-basal direction. Thus, spatial and temporal aspects of early corticogenesis are recapitulated and can be manipulated in this ESC culture.
Cerebellum Paper
ABSTRACT – Purkinje cells are the sole output neurons of the cerebellar cortex and their dysfunction causes severe ataxia. We found that Purkinje cells could be robustly generated from mouse embryonic stem (ES) cells by recapitulating the self-inductive signaling microenvironments of the isthmic organizer. The cell-surface marker Neph3 enabled us to carry out timed prospective selection of Purkinje cell progenitors, which generated morphologically characteristic neurons with highly arborized dendrites that expressed mature Purkinje cell–specific markers such as the glutamate receptor subunit GluRδ2. Similar to mature Purkinje cells, these neurons also showed characteristic spontaneous and repeated action potentials and their postsynaptic excitatory potentials were generated exclusively through nonNMDA glutamate receptors. Fetal transplantation of precursors isolated by fluorescence-activated cell sorting showed orthotopic integration of the grafted neurons into the Purkinje cell layer with their axons extending to the deep cerebellar nuclei and dendrites receiving climbing and parallel fibers. This selective preparation of bona fide Purkinje cells should aid future investigation of this important neuron.
Tissue Engineering the Optic Cup of the Eye
Nature – Self-organizing optic-cup morphogenesis in three-dimensional culture
Balanced organogenesis requires the orchestration of multiple cellular interactions to create the collective cell behaviours that progressively shape developing tissues. It is currently unclear how individual, localized parts are able to coordinate with each other to develop a whole organ shape. Here we report the dynamic, autonomous formation of the optic cup (retinal primordium) structure from a three-dimensional culture of mouse embryonic stem cell aggregates. Embryonic-stem-cell-derived retinal epithelium spontaneously formed hemispherical epithelial vesicles that became patterned along their proximal–distal axis. Whereas the proximal portion differentiated into mechanically rigid pigment epithelium, the flexible distal portion progressively folded inward to form a shape reminiscent of the embryonic optic cup, exhibited interkinetic nuclear migration and generated stratified neural retinal tissue, as seen in vivo. We demonstrate that optic-cup morphogenesis in this simple cell culture depends on an intrinsic self-organizing program involving stepwise and domain-specific regulation of local epithelial properties.
Tissue Engineering the Pituitary Gland
Nature – Self-formation of functional adenohypophysis in three-dimensional culture
The adenohypophysis (anterior pituitary) is a major centre for systemic hormones. At present, no efficient stem-cell culture for its generation is available, partly because of insufficient knowledge about how the pituitary primordium (Rathke’s pouch) is induced in the embryonic head ectoderm. Here we report efficient self-formation of three-dimensional adenohypophysis tissues in an aggregate culture of mouse embryonic stem (ES) cells. ES cells were stimulated to differentiate into non-neural head ectoderm and hypothalamic neuroectoderm in adjacent layers within the aggregate, and treated with hedgehog signalling. Self-organization of Rathke’s-pouch-like three-dimensional structures occurred at the interface of these two epithelia, as seen in vivo, and various endocrine cells including corticotrophs and somatotrophs were subsequently produced. The corticotrophs efficiently secreted adrenocorticotropic hormone in response to corticotrophin releasing hormone and, when grafted in vivo, these cells rescued the systemic glucocorticoid level in hypopituitary mice. Thus, functional anterior pituitary tissue self-forms in ES cell culture, recapitulating local tissue interactions
.
If you liked this article, please give it a quick review on ycombinator or StumbleUpon. Thanks
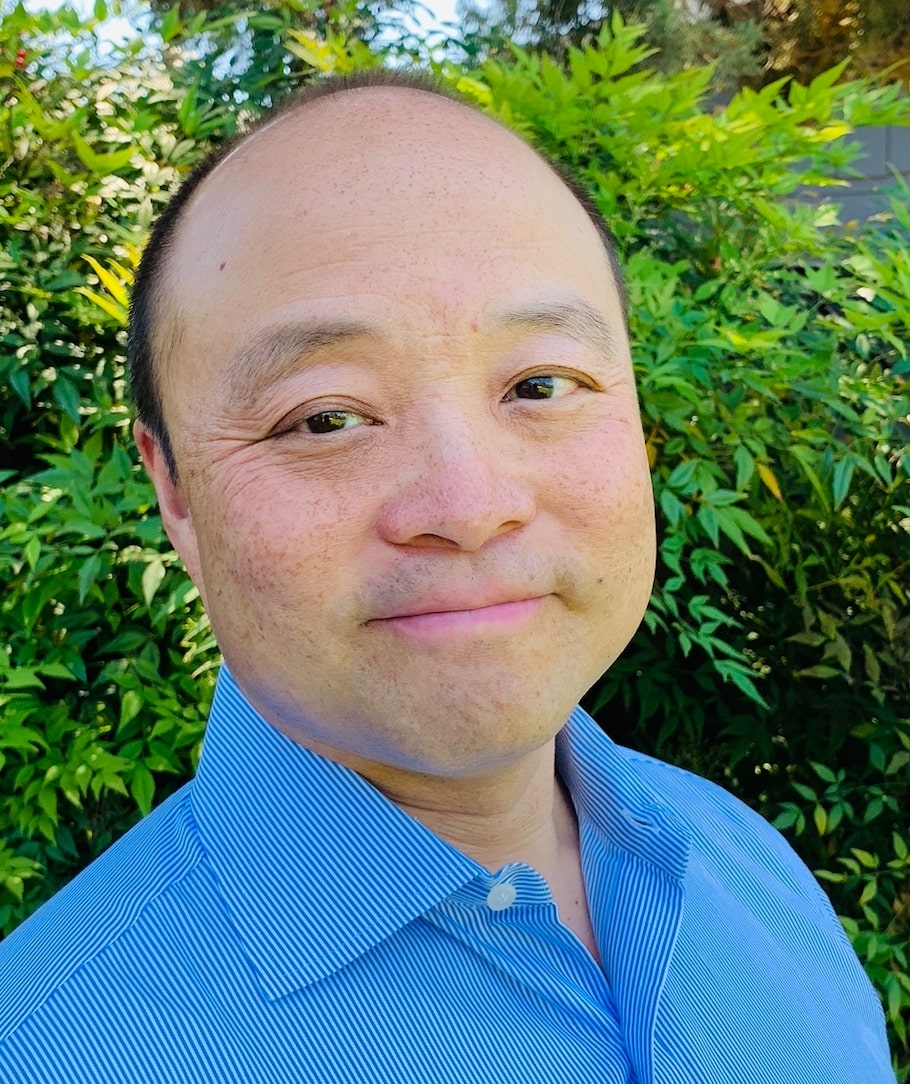
Brian Wang is a Futurist Thought Leader and a popular Science blogger with 1 million readers per month. His blog Nextbigfuture.com is ranked #1 Science News Blog. It covers many disruptive technology and trends including Space, Robotics, Artificial Intelligence, Medicine, Anti-aging Biotechnology, and Nanotechnology.
Known for identifying cutting edge technologies, he is currently a Co-Founder of a startup and fundraiser for high potential early-stage companies. He is the Head of Research for Allocations for deep technology investments and an Angel Investor at Space Angels.
A frequent speaker at corporations, he has been a TEDx speaker, a Singularity University speaker and guest at numerous interviews for radio and podcasts. He is open to public speaking and advising engagements.