Tiny, implantable computers that would restore brain function lost to disease or injury is the goal of University of Washington research recently funded by a $1 million, three-year grant from the W.M. Keck Foundation. The Keck project is the next step in advancing the technology of miniature devices developed at the UW to record from and stimulate the brain, spinal cord and muscles.
Dr. Eberhard E. Fetz, UW professor of physiology and biophysics and a core staff researcher at the Washington National Primate Research Center have successfully deployed tiny, battery-powered implantable brain-computer interfaces called neurochips in animals.
The neurochip can record nerve cell activity in one part of the brain, process this activity and then stimulate cells in another brain region. The battery-powered device operates continuously during free behavior. When primates carry out their usual daily activities – socializing, climbing, eating, and exploring – their brains can learn to exploit these new resources under normal behavioral conditions.
One potential clinical application is to bridge lost biological connections. For example, the researchers have shown that monkeys can learn to bypass an anesthetic block in the nerves of the arm and to activate temporarily paralyzed muscles with activity of cortical neurons. In some ways the device acts as a volition processor, tapping into signals representing the will to move and using them to stimulate the paralyzed muscles to reach targets.
“Using an implantable computer interface to implement novel interactions between brain sites opens many fundamentally new research directions,” Fetz said, “depending on the site of recording and stimulation, and how these signals are processed and transformed.”
He explained that a second application is to promote neural plasticity, which could strengthen connections and allow some of the brain’s functions to be rescued when impaired. This happens naturally when people recover the ability to move or speak again after a stroke or brain injury. The bidirectional brain computer interface could facilitate this recovery and exploit the brain’s innate talent for re-organizing itself as it heals.
“We expect that the recurrent type of brain computer interface we are trying to develop,” he added, “will eventually have numerous clinical applications for bridging damaged biological pathways and strengthening weak neural connections.” For example, signals from the motor-control regions of the brain can be used to stimulate parts of the spinal cord to evoke coordinated movements. This would create connections that could replace lost pathways between the brain and spinal cord, a loss that occurs with strokes and spinal cord injuries.
Many labs around the world are working on brain-computer interfaces that convert neural activity to control of external devices such as prosthetic limbs or computer cursors. What makes the recently funded project unusual is that its scientists are developing a recurrent implantable device that would interact bidirectionally with the brain. By operating autonomously and continuously, without the need for connection to external instrumentation, it would facilitate long-term behavioral adaptation and plasticity.
The proposed research plans to develop this new paradigm to promote restoration of brain, spine, and muscle function. The work could eventually lead to miniaturized electrical and biological interfaces that operate around the clock on a small amount of power while the wearer goes about his or her usual activities, according to Fetz. He added that, if successful, this implantable technology would advance the ability of subjects to effectively control a brain computer interface by allowing long-term adaptation to consistent contingencies, and would open opportunities for the brain to exploit bidirectional interactions with miniature computers. This implementation of continuous reciprocal interaction goes beyond the existing paradigm of using brain signals to control external devices through tethered connections.
As part of the project the team also plans to create a powerful multichannel “Keck Active Electrode Array” with integrated electronics to record and stimulate large numbers of brain sites. This array would operate with electrodes on the surface of the brain and be less invasive than penetrating intracortical electrodes.
May 7, 2011 there will be a talk on recurrent Brain computer interfaces
A prior talk in 2008 – The adaptive self and brain-computer interfaces
The conscious volitional self in our brain perceives and interacts with the world through sensory, motor and cognitive systems that involve largely subconscious neural mechanisms. Experimental manipulations of these mechanisms reveal the brain’s remarkable ability to adapt to changed conditions. The volitional self can also be extended through artificial devices, such as brain-machine interfaces, which exploit the brain’s ability to incorporate prosthetic extensions. Accurate control of brain machine interfaces depends on a combination of effective decoding algorithms and the brain’s ability to adaptively modify its neural activity. Recently developed implantable recurrent brain-computer interfaces provide artificial feedback connections that the brain can learn to incorporate and that can also modify the brain’s neural connections. This talk will explore these issues in light of current advances in neuroscience and neuroprosthetics.
Volitional control of neural activity: implications for brain–computer interfaces by Fetz (2007)
Successful operation of brain–computer interfaces (BCI) and brain–machine interfaces (BMI) depends significantly on the degree to which neural activity can be volitionally controlled. This paper reviews evidence for such volitional control in a variety of neural signals, with particular emphasis on the activity of cortical neurons. Some evidence comes from conventional experiments that reveal volitional modulation in neural activity related to behaviours, including real and imagined movements, cognitive imagery and shifts of attention. More direct evidence comes from studies on operant conditioning of neural activity using biofeedback, and from BCI/BMI studies in which neural activity controls cursors or peripheral devices. Limits in the degree of accuracy of control in the latter studies can be attributed to several possible factors. Some of these factors, particularly limited practice time, can be addressed with long-term implanted BCIs. Preliminary observations with implanted circuits implementing recurrent BCIs are summarized.
Continuous operation of a cortical recurrent BCI leads to long-lasting changes in physiological connections Top: intracranial microstimulation at 3 different motor cortex sites with the monkey at rest evoked 3 different muscle responses (centre) and different isometric torques about the wrist (right). Arrows at right indicate means of 200 ms torque trajectories. Middle: conditioning involved 2 days of triggering microstimuli at site Nstim for every spike recorded at Nrec during free behaviour and sleep. Bottom: after conditioning the output effects evoked from site Nrec had changed to include those from Nstim, an effect that lasted beyond a week. A plausible mechanism is Hebbian strengthening of synaptic connections from Nrec to Nstim
Brain Computer Interfaces: A Recurrent Neural Network Approach
This paper explores the use of recurrent neural networks in the field of Brain Computer Interfaces(BCI). In particular it looks at a recurrent neural network, an echostate network and a CasPer neural network and attempts to use them to classify data from BCI competition III’s dataset IVa. In addition it proposes a new method, EchoCasPer, which uses the CasPer training scheme in a recurrent neural network. The results showed that temporal information existed within the BCI data to be made use of, but further pre-processing and parameter exploration was needed to reach competitive classification rates.
The neurochip (2007) created by Jackson and his team has two circuit boards, a battery, and electrode connections, all housed in a 6-cm-diam titanium casing. The electronics consist of two programmable system-on-chips, which allow flexible analog and digital input and output with low power consumption. When the device is placed on a monkey’s head, the animal continues normal behavior. Data are recorded to a chip with 16-Mb nonvolatile RAM. The data are then downloaded offline using an infrared link. The device also has a constant-current source to electrically stimulate neurons.
Other researchers working on the project at the University of Washington include Jaideep Mavoori, PhD, who developed the device for his doctoral thesis, and Eberhard Fetz, PhD, professor of physiology and biophysics. Jackson will continue to work on the chip at the University of Newcastle upon Tyne, where he hopes to implant artificial connections between two different areas in the brain, as opposed to between two sites within the same area. He’ll also be collaborating with Fetz’s group in Washington to further develop the device.
If you liked this article, please give it a quick review on ycombinator or StumbleUpon. Thanks
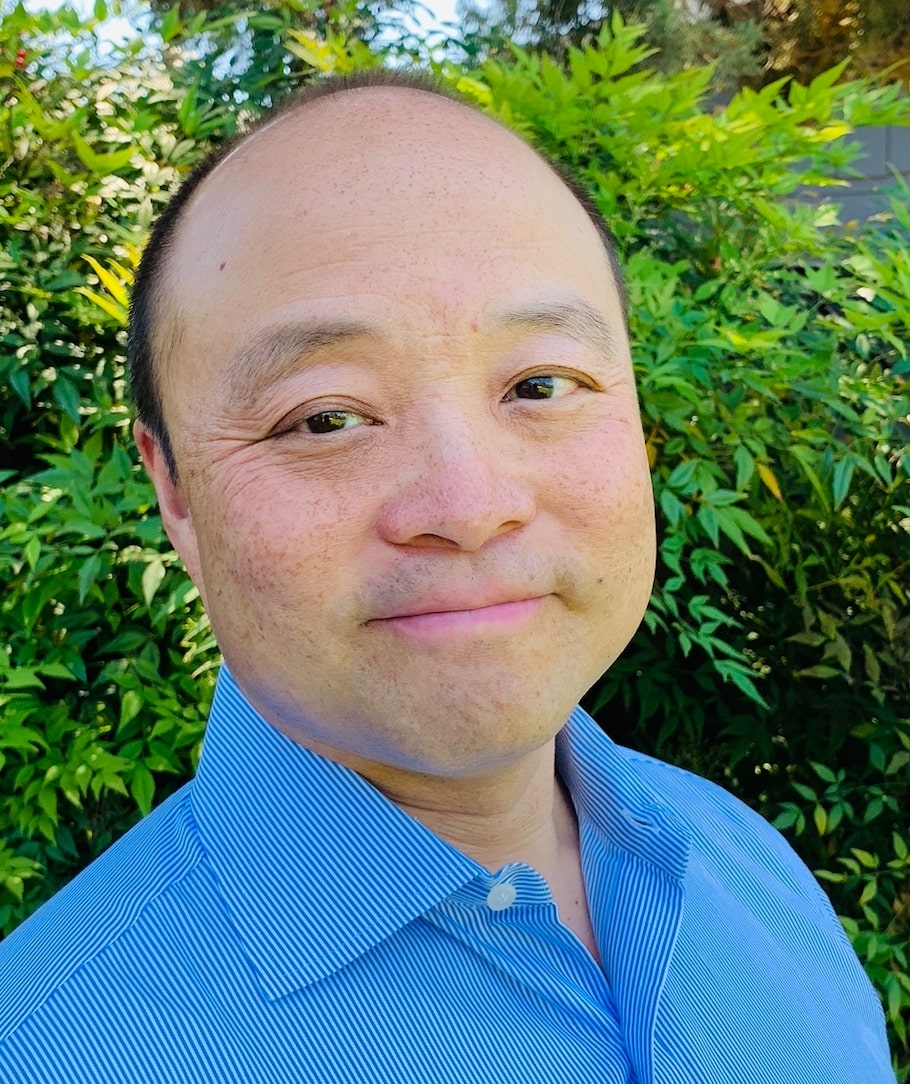
Brian Wang is a Futurist Thought Leader and a popular Science blogger with 1 million readers per month. His blog Nextbigfuture.com is ranked #1 Science News Blog. It covers many disruptive technology and trends including Space, Robotics, Artificial Intelligence, Medicine, Anti-aging Biotechnology, and Nanotechnology.
Known for identifying cutting edge technologies, he is currently a Co-Founder of a startup and fundraiser for high potential early-stage companies. He is the Head of Research for Allocations for deep technology investments and an Angel Investor at Space Angels.
A frequent speaker at corporations, he has been a TEDx speaker, a Singularity University speaker and guest at numerous interviews for radio and podcasts. He is open to public speaking and advising engagements.