Vikas Berry, William H. Honstead professor of chemical engineering, has developed a novel process that uses a diamond knife to cleave graphite into graphite nanoblocks, which are precursors for graphene quantum dots. These nanoblocks are then exfoliated to produce ultrasmall sheets of carbon atoms of controlled shape and size.
By controlling the size and shape, the researchers can control graphene’s properties over a wide range for varied applications, such as solar cells, electronics, optical dyes, biomarkers, composites and particulate systems.
Molecular dynamics snapshot of stretched graphene being nanotomed via a diamond knife.
“The process produces large quantities of graphene quantum dots of controlled shape and size and we have conducted studies on their structural and electrical properties,” Berry said.
While other researchers have been able to make quantum dots, Berry’s research team can make quantum dots with a controlled structure in large quantities, which may allow these optically active quantum dots to be used in solar cell and other optoelectronic applications.
“There will be a wide range of applications of these quantum dots,” Berry said. “We expect that the field of graphene quantum dots will evolve as a result of this work since this new material has a great potential in several nanotechnologies.”
It has been know that because of the edge states and quantum confinement, the shape and size of graphene quantum dots dictate their electrical, optical, magnetic and chemical properties. This work also shows proof of the opening of a band-gap in graphene nanoribbon films with a reduction in width. Further, Berry’s team shows through high-resolution transmission electron micrographs and simulations that the edges of the produces structures are straight and relatively smooth.
(a) A descriptive sketch of the nanotomy process showing the diamond-knife-based mechanical cleaving of HOPG block to produce GNBs for GNR production. Process parameters: dr=step-size and θv=angle of cleavage. (c) Sketch of the two-step nanotomy process to produce GNBs for GQD production. (b,d) GNRs and GQDs are produced by exfoliating the corresponding GNBs in chlorosulphonic acid (superacid). TEM micrographs of 30 nm GNRs and FESEM micrographs of GQDs of different shapes (square, rectangle and triangle).
Because of the edge states and quantum confinement, the shape and size of graphene nanostructures dictate their electrical, optical, magnetic and chemical properties. The current synthesis methods for graphene nanostructures do not produce large quantities of graphene nanostructures that are easily transferable to different substrates/solvents, do not produce graphene nanostructures of different and controlled shapes, or do not allow control of GN dimensions over a wide range (up to 100 nm). Here we report the production of graphene nanostructures with predetermined shapes (square, rectangle, triangle and ribbon) and controlled dimensions. This is achieved by diamond-edge-induced nanotomy (nanoscale-cutting) of graphite into graphite nanoblocks, which are then exfoliated. Our results show that the edges of the produced graphene nanostructures are straight and relatively smooth with an ID/IG of 0.22–0.28 and roughness less than 1 nm. Further, thin films of GN-ribbons exhibit a bandgap evolution with width reduction (0, 10 and ~35 meV for 50, 25 and 15 nm, respectively).
GNRs and GQDs of controlled shape and size.
GNs were synthesized, via a versatile nanotomy process, with high structural control (shapes: squares, rectangles, ribbons and triangles; and size: adjusted at 5 nm resolution) and colloidal monodispersivity. The GNs are exfoliated from GNBs produced via nanotomy of HOPG. This highly versatile process can produce a variety of GN structures. Further, the Raman and the HRTEM measurement show that the GNs had relatively smooth edges; while the MD simulations and HRTEM micrographs show that the edges are predominantly zigzag. Our electrical studies show evolution of bandgap of thin GN films with GNR’s width. We envision that this versatile process can provide access to a wide variety of GNs at large densities (on substrates or as dispersions) for development of fundamental optical/electrical/structural correlations and novel applications. Further, the nanotomy process may be applied to other 2D nanomaterials (BN, MoS2 and NbSe2) to produce unique 2D nanostructures, which can significantly expand the scope of their applications and fundamental studies.
24 pages of supplemental information
If you liked this article, please give it a quick review on ycombinator or StumbleUpon. Thanks
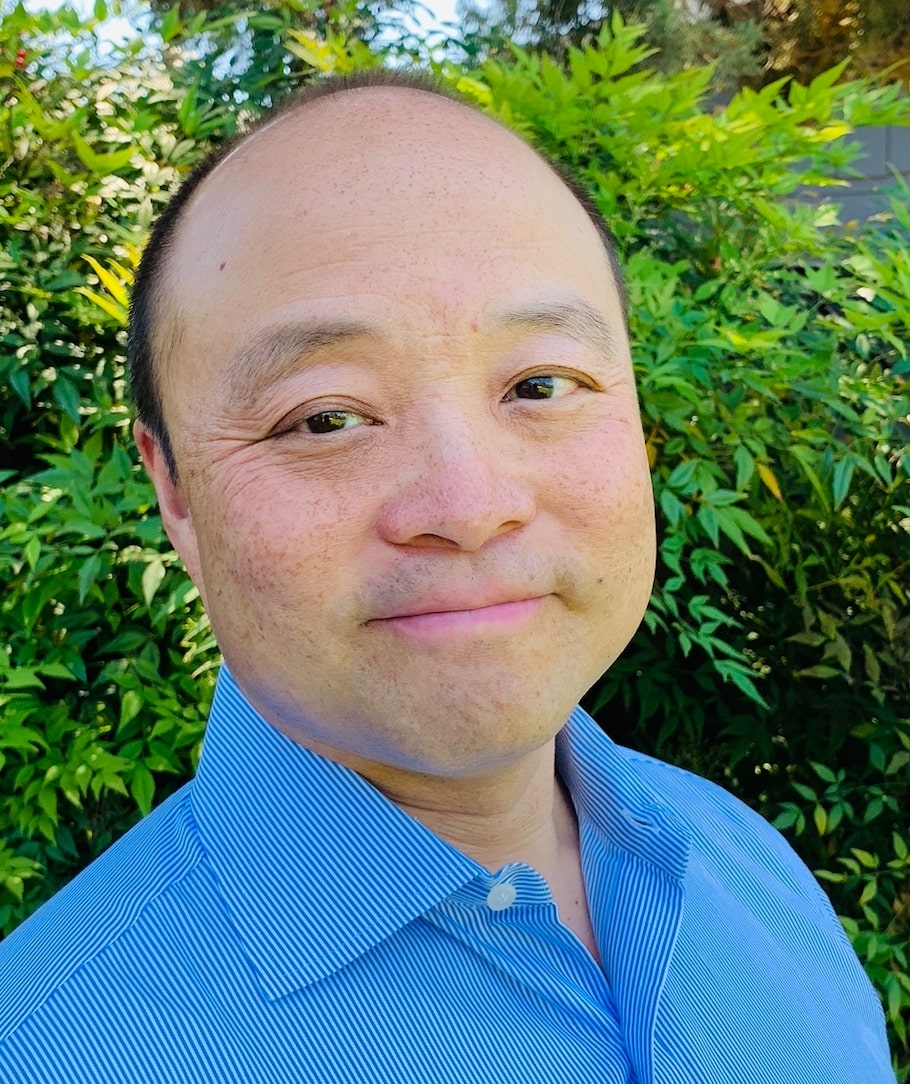
Brian Wang is a Futurist Thought Leader and a popular Science blogger with 1 million readers per month. His blog Nextbigfuture.com is ranked #1 Science News Blog. It covers many disruptive technology and trends including Space, Robotics, Artificial Intelligence, Medicine, Anti-aging Biotechnology, and Nanotechnology.
Known for identifying cutting edge technologies, he is currently a Co-Founder of a startup and fundraiser for high potential early-stage companies. He is the Head of Research for Allocations for deep technology investments and an Angel Investor at Space Angels.
A frequent speaker at corporations, he has been a TEDx speaker, a Singularity University speaker and guest at numerous interviews for radio and podcasts. He is open to public speaking and advising engagements.