The economics of SMRs challenges the widely held belief that nuclear reactors are characterized by significant economies of scale.
According to the standard scaling function, the hypothetical overnight cost (per unit of installed capacity) of the SMR-based power station will be 74 percent higher compared to a single large-scale reactor. Based on various studies in the literature, the authors posit that the combined impact of multiple units and learning effects is a 22 percent reduction in specific capital costs for the SMR-based station. To quantify the impact of construction schedule, the authors assume that the construction times of the large reactor and the SMR units are five and three years respectively. The shorter construction duration results in a 5 percent savings for the SMRs. Temporal flexibility (four sequentially deployed SMRs with the first going into operation at the same time as the large reactor and the rest every 9 months thereafter) and design simplification led to 5 and 15 percent reductions in specific capital costs respectively for the SMRs. When all these factors are combined, the SMR-based station suffers a specific capital cost disadvantage of only 4 percent as compared to the single large reactor of the same capacity.
For the plants below 300 MW(e) the overnight capital costs are below US$ 1 billion. Small absolute overnight capital costs make the SMRs attractive to a broader range of investors, including a variety of private companies (not necessarily affiliated with nuclear sector) and the utilities whose own funds are insufficient to finance a large reactor project. China’s HTR-PM has estimated overnight capital costs of less than $317 million. China’s large reactors are at or below the cost that is provided for the South Korean nuclear reactors. The $4.2 billion quote for the Korean reactors were for two large 1.34 GWe reactors.
By and around 2020, about 11 SMRs developed in Argentina, China, Republic of Korea, Russian Federation and the United States could be deployed as first-of-a-kind plants in their countries of origin. In case of success, these reactors could later be considered for export to developing countries starting from the mid-2020s.
SMRs would provide for greater siting flexibility and be a better fit for many developing countries with small electrical grids where they could facilitate incremental growth of the grid.
All current SMRs designs are expected to be deployed first in their countries of origin or in another developed technology holder country. Such plants would need to operate for several years before they are offered for export to developing countries.
Small modular reactors have compact designs—e.g., the containment vessels of 25 Westinghouse SMRs (225 MW(e) each) could fit into a single AP-1000 containment vessel—and could be manufactured in factories or other central facilities and then transported (along with the necessary containment walls, turbines for generating electricity, control systems, and so on) to the site of a future plant by track or rail. Building reactors in a factory could substantially decrease construction times and lead to savings on both construction and financing costs.
China HT-PMR
The traditional HTGR designs are employing the direct gas-turine Brayton cycle offering high energy conversion efficiency—up to 55% as compared to 32% in PWR. They incorporate provisions for multiple co-generation applications, such as hydrogen production and seawater desalination. However, deployment of these designs, which include the the Japanese GTHTR300, the Russian-U.S. GT-MHR, and the U.S. NGNP, is being anticipated within the timeframe of 2025 and beyond. One design that seems to be ready for deployment is the Chinese HTR-PM. The HTR-PM, uses he concept of a moveable pebble bed fuel (wherein the TRISO coated particles are embedded in graphite balls that move along the annular core in reactor operation) that is similar to that of PBMR (design development for PBMR has been stopped in 2010 owing to a financial collapse of the South African PBMR Pty). However, it employs an indirect cycle with superheated steam in the power circuit. The Rankine cycle that is being employed with multiple reheats of steam secures plant efficiency of about 42%. The HTR-PM provides for no non-electrical applications and is deemed for electricity production within a standard three module plant. It’s design is backed by a decade long operation of a 10 MW(th) HTR-10 prototype at the Tsinghua University in China.
Lead Bismuth Reactors
Lead and lead-bismuth eutectic cooled reactors could be considered together since they employ similar technologies—e.g., coolant purification and control of corrosion for both coolants are similar, although the details are different. Regarding implementation, progress has been achieved in the lead-bismuth eutectic reactors in Russia. Presently, there are no lead cooled small modular
reactors under development anywhere in the world, so this section is restricted to lead-bismuth SMRs.
The SVBR-100 design is backed by 80 reactor-years of operating experience of the propulsion reactors in the seven Russian Alpha-class nuclear submarines. In addition to the resolution of the corrosion problem, the Russian submarine program had succeeded in resolving the problem of volatile 210Po trapping and developed a safe freezing/defreezing procedure for the lead-bismuth coolant (Polonium-210 is a strong alpha emitter that is lethally toxic to human beings if inhaled or digested; 210 Po is generated from 209Bi under irradiation and has a half-life of ~138 days). Like all liquid metal cooled reactors, SVBR-100 operates at near-atmospheric pressure. As the coolant based on lead-bismuth eutectic is chemically inert in water and air, the plant has no intermediate heat transport system. The compact SVBR-100 module is immersed in a refillable pool with water at atmospheric pressure. Boiling of water in the pool helps remove the heat from the reactor vessel outer surface in accidents.
The SVBR-100 can operate with different types of nuclear fuel. For the near-term, uranium-dioxide based fuel load is considered with an average uranium enrichment of 16.3% by weight. The reactor is designed for continuous operation on the site in the course of 7–8 years, after which whole core refulling is performed on site. When operated in a closed nuclear fuel cycle, SVBR-100 will retain the effective fissile mass in the core, i.e., will require no additional fissile materials to be added at a refueling.
The GEN4 Energy Module (formerly known as the Hyperion Power Module) shares many common features with the SVBR-100. However, in contrast to the SVBR-100, it employs natural circulation of the primary coolant in normal operation mode—the SVBR-100 uses pumps for that purpose. Moreover, it is designed to be fueled and de-fueled at factory—the SVBR-100 design provides for whole-core on-site refueling). The GEN4 Energy design is based on the results of R and D carried out at the Los Alamos National Laboratory. Like SVBR-100, it is assumed to be used within single- or multi-module nuclear power plants.
No matter how early the first-of-a-kind, non-commercial SVBR-100 or GEN4 Energy Module plants will be deployed, the long refueling intervals provided for by their designs (5-15 years) will necessitate long testing and demonstration programs. Thus, their promotion as exportable commercial products cannot be realistically expected before 2025.
If you liked this article, please give it a quick review on ycombinator or StumbleUpon. Thanks
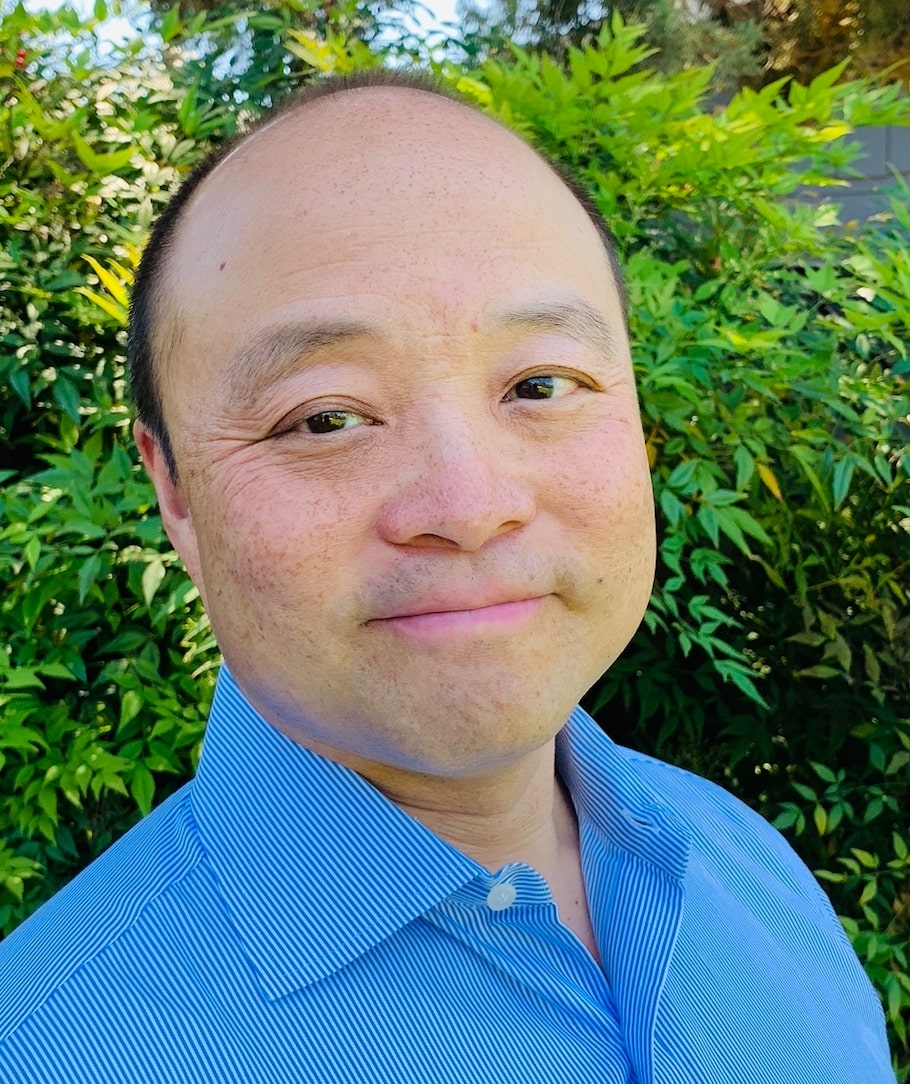
Brian Wang is a Futurist Thought Leader and a popular Science blogger with 1 million readers per month. His blog Nextbigfuture.com is ranked #1 Science News Blog. It covers many disruptive technology and trends including Space, Robotics, Artificial Intelligence, Medicine, Anti-aging Biotechnology, and Nanotechnology.
Known for identifying cutting edge technologies, he is currently a Co-Founder of a startup and fundraiser for high potential early-stage companies. He is the Head of Research for Allocations for deep technology investments and an Angel Investor at Space Angels.
A frequent speaker at corporations, he has been a TEDx speaker, a Singularity University speaker and guest at numerous interviews for radio and podcasts. He is open to public speaking and advising engagements.