A number of commercial high-temperature reactors are under development around the world. But this year, a consortium of petrochemical companies and reactor manufacturers agreed to back the Antares high-temperature reactor design from the French company AREVA, based in Paris. “All that’s left is about $800 million of work design and licensing effort required to get the technology to the point where the Nuclear Regulatory Commission could approve it,” says Fred Moore, head of the division that provides power and steam for the Dow Chemical Company, headquartered in Midland, Michigan. He estimates that this should take 5–7 years. If all goes to plan, high-temperature systems will be among the first advanced reactors to be deployed, starting in the 2020s.
Not far behind would be fast reactors, which tackle a problem that high-temperature reactors cannot: spent nuclear fuel. Fast reactors could consume the stuff, turning waste into energy and easing the disposal problem.
Fission neutrons are ‘fast’ when they have just emerged from newly split nuclei at a mean energy of roughly 2 million electronvolts. In light-water reactors, collisions with the hydrogen nuclei in the coolant water quickly slow the neutrons to just a fraction of an electron volt, which makes them more likely to trigger another fission reaction. But slow neutrons have a drawback: instead of splitting the target uranium nuclei, they often get absorbed, transforming the nuclei into long-lived isotopes of plutonium, neptunium, americium, curium or other heavy elements — the ones that collectively make disposal of spent fuel a nightmare. Fast neutrons, by contrast, rarely get absorbed. They don’t hit their targets often, but when they do, that target almost always splits. As a result, fast reactors not only avoid the problem of producing long-lived isotopes, but can even destroy them in spent fuel.
Molten-salt reactors
The great virtue of solid reactor fuel is its predictable geometry. The great drawback is its complexity. The intensity of neutron bombardment, the distribution of fission products, the radiation damage to the fuel’s crystalline structure: everything varies from point to point. This is a constant headache for designers trying to ensure that the reactor operation is stable — and trying to convince regulators that even the worst meltdown won’t allow any part of the fuel to collapse into a critical mass.
But all these concerns go away when the fuel is already a liquid — one major reason why Oak Ridge wanted to develop the molten-salt reactor back in the 1960s. ‘Molten-salt’ refers to the fuel, usually uranium tetrafluoride, which is liquid at operating temperatures when blended with ‘FLiBe’: a mixture of lithium fluoride and beryllium fluoride that serves as a coolant. “It’s a pot — a big, dumb, pot,” says Forsberg. “You throw fuel in, it’s mixed, and the overall composition changes not at all.”
Liquid fuel has another big advantage, says Sorensen: “You don’t have to remove it from the reactor until it’s completely consumed.” Instead, the fuel is circulated through an external recycling unit that extracts the fission products continuously, keeping the fuel from being poisoned. The design also allows for an elegant approach to safety, says Sorensen: at the bottom of the reactor is a hole, plugged with a chunk of fuel that is kept solid by a refrigeration unit. If the reactor loses power in an emergency, the refrigeration will cease, the plug will melt and the fuel will safely drain into underground holding tanks. Finally, the molten-salt design can accommodate a variety of fuels, ranging from conventional uranium to raw nuclear waste or thorium — an element that is roughly three times more abundant than uranium.
For all of that, reviving the molten-salt reactor after a four-decade hiatus is a daunting task. “We have to rebuild a knowledge base that has largely gone away,” says Sorensen. He founded Flibe Energy to try, though. The company is developing a 40-megawatt reactor that might be used on military bases so that they can operate independently of the grid.
Solid chance
In September 2011, Forsberg, Peterson, MIT’s Lin-wen Hu and Todd Allen, a nuclear engineer at the University of Wisconsin-Madison, became principal investigators on a 3-year, DOE-funded project that could be a step on the way to the molten-salt reactor: a FLiBe-cooled high-temperature reactor. “No one has ever built a salt-cooled solid-fuel reactor,” says Peterson. But if the project works, the reactor core could be four to five times smaller than those in other designs and, because of the stability of the FLiBe salt, it would “always be hundreds of degrees below the failure limits”, he says.
Peterson says that the company could have a test reactor within a decade, although “that assumes abundant resources”. That is a big assumption: the global economic crisis has made financing for all advanced reactors much harder to come by. Furthermore, notes Corradini, pointing to the sudden abundance of shale gas in the United States, “cheap fossil fuels have postponed many of the clean-energy projects in the United States, not just nuclear”.
Paul Genoa, director of policy development for the Nuclear Energy Institute trade group in Washington DC, takes the long view. “We did the light-water reactors first, to get going,” he says. Next, in the 2020s, will come advanced light-water reactors for increased safety, followed closely by high-temperature reactors that expand the attack on carbon emissions. “And then we build fast reactors to consume the waste.”
Molten-salt reactors are something of a wild card, says Genoa, but are worth developing. Some even wilder cards are under investigation: one notable example is the accelerator-driven reactor, which would drive fission reactions using neutrons from a high-energy particle accelerator. It could be fuelled with thorium, and shut down instantly by switching off the accelerator.
But will nuclear energy really evolve? Those in the field see reason for optimism, particularly if the increasingly tangible consequences of climate change force governments to put a price on carbon. Even the Fukushima disaster could ultimately spur new nuclear technologies, says Genoa. “It scared people and made them concerned about nuclear energy,” he says. But as people looked more closely, “they said, ‘Hey, those were 30-year-old plants’”. In time, he says, smart, new reactors will look a whole lot more appealing.
If you liked this article, please give it a quick review on ycombinator or StumbleUpon. Thanks
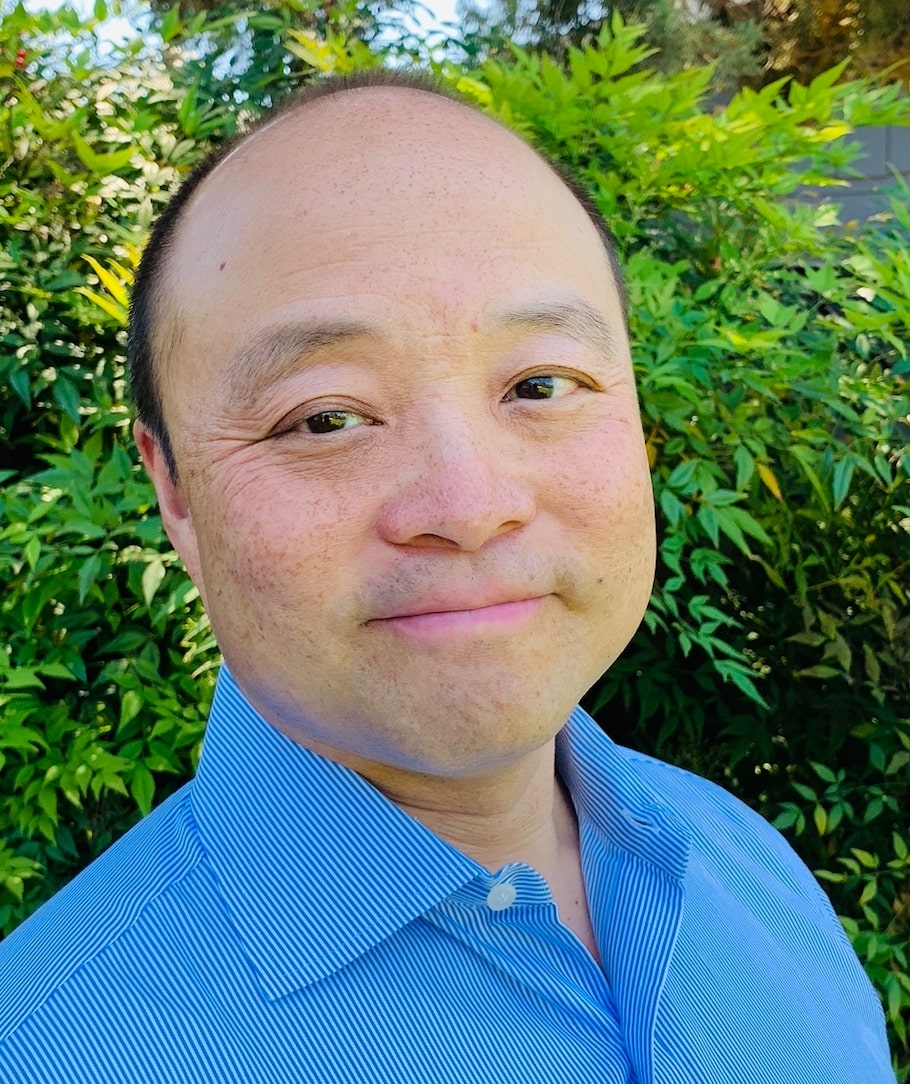
Brian Wang is a Futurist Thought Leader and a popular Science blogger with 1 million readers per month. His blog Nextbigfuture.com is ranked #1 Science News Blog. It covers many disruptive technology and trends including Space, Robotics, Artificial Intelligence, Medicine, Anti-aging Biotechnology, and Nanotechnology.
Known for identifying cutting edge technologies, he is currently a Co-Founder of a startup and fundraiser for high potential early-stage companies. He is the Head of Research for Allocations for deep technology investments and an Angel Investor at Space Angels.
A frequent speaker at corporations, he has been a TEDx speaker, a Singularity University speaker and guest at numerous interviews for radio and podcasts. He is open to public speaking and advising engagements.