Direct Fusion Drive, is a unique fusion engine concept based on the Princeton Field-Reversed Configuration (PFRC) fusion reactor under development at the Princeton Plasma Physics Laboratory. The truly game-changing levels of thrust and power in a modestly sized package could integrate with our current launch infrastructure while radically expanding the science capability of these missions. NIAC grants require that a technology be studied in the context of a specific mission. The mission context is the delivery of a Pluto orbiter with a lander, which cannot be done with any other technology. Direct Fusion Drive (DFD) provides moderate thrust to allow for reasonable transit times to Pluto while delivering substantial mass to orbit: 1000 kg delivered in four years using 5 N constant thrust.
Since DFD provides power as well as propulsion in one integrated device, it will also provide as much as 1 MW of useful electrical power to the payloads upon arrival. This enables high-bandwidth optical communication, powering of the lander from orbit, and radically expanded options for instrument design.
The Princeton Field-Reversed Configuration (PFRC), which is a steady-state fusion reactor concept with heating via rotating magnetic fields. A thrust model of the reactor is presented that is based on a fluid code modeling the exchange of energy in the plasma surrounding the reactor.
The Direct Fusion Drive concept is an extension of ongoing fusion research at Princeton Plasma Physics Laboratory dating to 2002. The Princeton Field-Reversed Configuration machine (PFRC) employs a unique radio frequency (RF) plasma heating method invented by Dr. Samuel Cohen. Odd-parity heating was first theorized in 2000 and demonstrated in the 4 cm radius PFRC-1 experiment in 2006. Experiments are ongoing with the second-generation machine, PFRC-2, which has a plasma radius of 8 cm. Studies of electron heating in PFRC-2 have surpassed theoretical predictions, recently reaching 500 eV with pulse lengths of 300 ms, and experiments to measure ion heating with input power up to 200 kW are ongoing.
When scaled up to achieve fusion parameters, PFRC would result in a 4-8 meter long, 1.5 meter diameter reactor producing 1 to 10 MW. This reactor would be uniquely small and clean among all fusion reactor concepts, producing remarkably low levels of damaging neutrons.
The plasma heating method uses a unique configuration of the radio antenna. Attempts have been made to heat FRC plasmas with RF before, but always with single-loop antenna that resulted in a near-FRC plasma but with open field lines. They call this even-parity heating due to the symmetry of the induced magnetic field. Open field lines give the plasma an opportunity to escape and reduce confinement time. In contrast, the PFRC antenna are figure-8s. Two pairs operate 90 degrees out of phase on adjacent sides of the plasma. An antenna (wrapped in orange Kapton tape) is clearly visible on the side of PFRC-2 . This results in so-called odd-parity heating – the magnetic field on one side of each figure-8 is in the opposite direction as the other side – and closed field lines in the generated FRC. Closed field lines keep the plasma trapped as it is heated. The oscillation of the currents in the RF antenna result in a rotating magnetic field, RMF, with about 0.3% of the strength of the axial magnetic field.
Reduction in neutrons is achieved through multiple channels. First and foremost, our choice of Helium-3 (3He) and deuterium (D) fuels results an aneutronic primary reaction. However, there are still D-D side reactions, the proportion of which is governed by the fusion cross-section at the relevant ion temperature.
The D-D side reactions are divided equally into two, a D-D reaction producing a 2.45 MeV neutron and a D-D reaction producing tritium (T). This tritium can then fuse with deuterium to produce a high energy 14.7 MeV neutron.
The PFRC is specifically designed to produced the absolute minimum number of neutrons from these side reactions.
1. the small size of the reactor results in a favorable ratio of surface area to plasma volume. reducing the wall load compared to larger machines.
2. they adjust the operating fuel ratio of 3He:D to 3:1, sacrificing some power density for lower radiation.
3. the reactor is designed to rapidily eliminate the tritium produced by the D-D side reactions, preventing any D-T reactions from occurring. This means that the only neutrons produced are those with energy of 2.45 MeV.
4. A reduction in neutrons may occur due to preferential heating of the 3He over the D by the rotating magnetic fields; at the correct frequency, they estimate that the 3He may reach a temperature of 140 keV while the D is only at 70 keV. This would result in another (CHECK) 10 fold reduction in neutrons. The final result of these design features is that only 1%, or perhaps even only 0.5% or less, of the fusion power is in 2.45 MeV neutrons, and the power in 14.7 MeV neutrons is effectively zero.
Initial analysis of the Pluto mission relied on simple Lambert trajectories that assumed relatively short acceleration and deceleration phases at the beginning and end of the trajectory. The power level is an input, along with a specific power and thrust efficiency, which then determines the Isp given a thrust level. This simple analysis indicated that assuming a fairly conservative specific power of 0.7 kW/kg, a mission was possible under 8000 kg using two 1 MW engines. A single SpaceX vehicle or Delta Heavy could launch the mission.
The mission would deliver 1000 kg to Pluto and then provide about 1MW of electrical power on orbit. The mission parameters assuming a 74 km/s transfer, which is possible for a launch date in 2036.
A burn time of 292 days corresponds to a required thrust of 16.2 N and an Isp of 12,554 seconds, assuming an overall thrust efficiency of 0.5.
Critical subsystems
include the superconducting coils, space radiators, cryogenic systems, high-power optical transmission systems, and thermal conversion. Available data suggests that research in all these areas has made tremendous
progress recently and we have not identified any roadblocks.
They received a Phase II NIAC grant in May, 2017 to continue this work, and also two NASA STTRs relating specifically to the RF subsystem and superconducting coil subsystem. We will be further refining our models and designs to create a roadmap for building the first DFD prototype. Ongoing work at PPPL on proving ion heating using the RMFo method in PFRC-2 will need to be followed by a larger machine with superconducting coils that can heat the plasma sufficiently to demonstrate fusion. We are actively searching for funding mechanisms to continue this research.
A compact 1 MW space power plant would be truly game-changing for multiple applications. In Earth orbit, it enables high-power payloads like radar as well as nearly unlimited orbit maneuverability.
In cislunar space, DFD could transform the envisioned Deep Space Gateway while also powering human bases on the lunar surface. Robotic missions to asteroids, Jupiter and its moons, and any other deep space destination become more faster, cheaper, and can return orders of magnitude more science. There are many missions that can be accomplished now with a small amount of helium-3 from terrestrial sources, and enormous reserves are available on the moon for future missions.
Under IR&D, they are developing Space Rapid Transit (SRT), a two stage to orbit launch vehicle that takes off and lands horizontally. SRT has an air-breathing first stage and an LH2/LO2 propelled second stage.
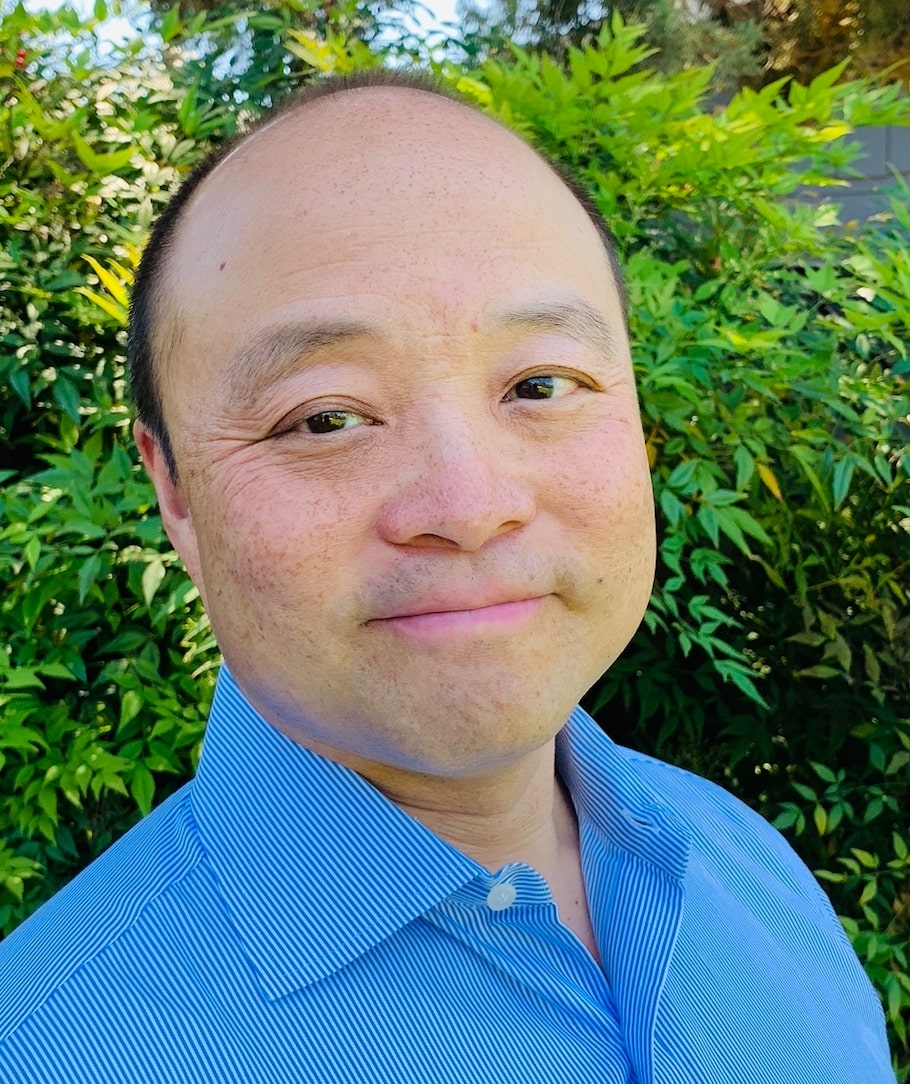
Brian Wang is a Futurist Thought Leader and a popular Science blogger with 1 million readers per month. His blog Nextbigfuture.com is ranked #1 Science News Blog. It covers many disruptive technology and trends including Space, Robotics, Artificial Intelligence, Medicine, Anti-aging Biotechnology, and Nanotechnology.
Known for identifying cutting edge technologies, he is currently a Co-Founder of a startup and fundraiser for high potential early-stage companies. He is the Head of Research for Allocations for deep technology investments and an Angel Investor at Space Angels.
A frequent speaker at corporations, he has been a TEDx speaker, a Singularity University speaker and guest at numerous interviews for radio and podcasts. He is open to public speaking and advising engagements.
Rotating magnetic field heating is a pipe dream, how will they keep heating and compressing at the same time to scale with energy, how much precice power electronics and feedback would you need. RF heating has. Its own problems. None of that will work. Neutral beams are too bulky. All in all, I don’t need to mention the meager thrust in this model, it ends up being a fun concept we considered and realized that won’t work.
Why not to use an induction of direct current generation taking advantage of the electrical charged plasma flux, instead of implement a complex Brayton cycle with its radiators and all the gear?
This is a misunderstanding of what they are actually doing. Some popular media articles look at technical papers and forward looking statements and then pick out the most “exciting” parts to make big headlines.
I know the people behind the DFD and they are good and solid people, who are excited about their work (and they should be, it is great!).
Ah, so you are trolling because you have an axe to grind. Got it!
As much as I love research, and think their goals should be high priority, this report and video are disturbing. Graphs labeled “Data” with energies up to 6MW for a 0.2MW experiment. Claims of making power for an experiment that at best returns a fraction of energy input. Another commenter who seems very familiar will disagree, but that setup does not look like they have the sheilding to attempt actual fusion (yet). For instance I would be skeptical that they have a sample of 3He in the lab at all. Society respects scientists and engineers because there is an expectation that they tell you things that are true. This lab and their Pr firm undermine that trust. If it were my organization I would want to continue the work, but groom potential replacements for the investigators on grounds of professional ethics. Of course this perception is based only on what is presented here, but there are clearly strategies of deception being employed. I expect to be castigated as not understanding, but really look at what they say and show. Integrity, this Pr firm would be a great fit for Mark Jacobson 😉
Sure, PPPL is a household name to anyone who is informed about plasma physics, especially here in New Jersey, where I live. Personally, I have an axe to grind with Princeton because this libtarded institution has forsaken fission. There isn’t a school in NJ with a real** nuclear program, which means that I cannot formally continue my education without attending a school 250 miles away.
A couple of million dollars charity funding doesn’t make it less of a hobby reactor.
I read this as: you expect to be paid to fiddle with magnets because you’re a plasma physicist. Plasma physicists find work in Silicon Valley designing machines to etch wafers.
Well, Leonardo da Vinci paid the bills with real work and managed to dream about pie in the sky BS on his own time.
**fission is real; fusion is pie in the sky money pit
For the Pluto mission, they would only use 0.5 kg of He3. The rest is D2.
Not much. 134kg, according to their NIAC Phase 2 paper.
That is because the electricity generation is only for the systems and not the propulsion.
I agree that 1g is not needed for an interplanetary mission. 0.1g would be more than enough. 1g+ would be nice for SSTO, though.
Falcon 9 1st stage thrust is 7600 kN, with a sea level Isp of 282 s (per Wikipedia). 7.6e6 * 282 * 9.8 = 21 GW of propulsive power.
Flow rate is 7.6e6 / (282 * 9.8) = 2750 kg/s.
Google says the fuel ratio is 2.3:1, so ~1920 kg/s oxygen and ~830 kg/s RP-1 kerosene (EDIT: mixed them up; fixed).
Their specific heat capacities are ~1 kJ/kg*K and ~2 kJ/kg*K, respectively (varies with temperature etc, but approximate is fine). The burn temperature is 3670 K.
That actually adds up to well above the extra 5 GW. Maybe it doesn’t heat up quite that much, or I’m missing some other detail.
Point is, almost all of the energy is carried away by the exhaust, most as propulsive power, and the rest as heat. Then that heat is dissipated as the exhaust cools. Only a small portion of the heat is transferred to the various structures. Same with NERVA (probably).
BUT, that assumes you can afford enough fuel flow to carry away all the heat, and don’t have any other systems that generate waste heat, and you only ever produce power and thrust simultaneously. In a realistic space ship, all of those assumptions may not hold. So you’ll still have non-propulsive waste heat remaining, that you’d still need to radiate away. Though maybe not 100 MW of it.
Even without the radiators, 10 tons isn’t much for an interplanetary mission, especially if it’s manned. But then again, 1 gee acceleration isn’t necessary either. We can make do with much less.
Great calculation!
From what I understand, NERVA reactors did not need heavy radiators at all and neither do chemical rocket engines. Some of those can get very high power. Falcon 9 produces a total power of 26 GW at liftoff. I am sure not all of that is converted into thrust. Yet, the engines are not melting but can be reused many times without issue.
Hydrogen has the highest heat capacity of any substance, at 14.3 kJ/(kg*K), but even with that, we’re only talking 14 MJ/kg if you let it heat up by 1000 K. Its heat of vaporization adds another ~0.5 MJ/kg. You’d need to pump almost 70 kg/s just to remove 1 GW of waste heat. That’s already a lot – compare that to their stated mass flow of less than 0.1 kg/s (IIRC). But we’re talking TWs of waste heat.
Direct conversion is nice, but then the next problem is running that much juice through the electrical system. Even superconductors have their limits.
The pulsed operation is a good point, but the question is which fraction of time is the reactor on? If it generates 2 TW of waste heat for even 1/100th of the time, that’s still 20 GW on average.
At the end, it’s the average power that determines the maximum performance. Let’s say optimistically that they somehow manage using all the tricks in the book to get it to 1 GW of propulsive power, 100 MW of non-propulsive waste heat, and 1000 s avg Isp. That should give them 100 kN of thrust. Those would be reasonable numbers, more or less. But difficult to achieve. But it still may not be enough for 1 gee acceleration – those 100 MW radiators would be heavy (plus the reactor, plus the fuel, plus all the other systems).
https://www.science.gov/topicpages/w/web+growth+machine.html
Antimatter rockets: the future of interstellar travel
[ all.antiquated.technology ]
Interstellar Travel: Approaching Light Speed
https://cosmosmagazine.com/technology/antimatter-ion-drives-nasas-plans-deep-space-propulsion
http://calistry.org/calculate/latticePlanesMillerIndices
https://www.worldscientific.com/doi/abs/10.1142/S0217732304016019
https://newatlas.com/flying-motorcycle-jetpack-aviation-speeder/58752/
video
https://www.youtube.com/watch?v=mTnU9W08qgk
http://www.xtal.iqfr.csic.es/Cristalografia/parte_03-en.html
I suppose that they could use some regenerative cooling, like chemical rocket engines use. The extra propellant flow would cool the engine. That super heated hydrogen could be exhausted out the back for a lot of extra thrust.
You do not need an Isp of 360,000 seconds anyway. An Isp in the low thousands would already be a game changer.
Also, I think that they are assuming some sort of direct conversion for recovering some of the energy for recharging the reactor for the next shot.
Also worth noting that the reactor is pulsed. So it can be started and stopped quickly for the purpose of letting it cool.
Maybe fire a few “shots”, then use the regenerative cooling (sort of like a NERVA) to provide thrust during the break. Then repeat.
Would have an Isp of ~1000 while it is cooling off and the higher Isp only while the reactor is running. Even an Isp of 10,000 or so on average would be amazing, especially with the high thrust that this could achieve from the NERVA type super heated hydrogen propulsion.
Taking a better look at that table, with D-He3 they’re claiming: Pf = 3.3e12 W, ve = 3.5e6 m/s, F = 3.3e5 N.
Notice that they give the Isp in terms of exhaust velocity, so no need to multiply by g0. So:
P = F * ve = 3.3e5 N * 3.5e6 m/s = ~1.16e12 W
Isp = ve / g0 = 3.5e6 m/s / 9.8 m/s^2 = ~3.6e5 s = ~360000 s
Thrust efficiency = 1.16e12 W / 3.3e12 W = ~35%
Waste heat = 3.3e12 W – 1.16e12 W = ~2.14e12 W = ~2.1 TW
With pB11, they claim: Pf = 9.9e12 W, ve = 1.3e6 m/s, F = 6.8e5 N.
P = F * ve = 6.8e5 N * 1.3e6 m/s = ~8.8e11 W
Isp = ve / g0 = 1.3e6 m/s / 9.8 m/s^2 = ~1.3e5 s = 130000 s
Thrust efficiency = 8.8e11 W / 9.9e12 W = ~9% (BAD!)
Waste heat = 9.9e12 W – 8.8e11 W = ~9e12 W = ~9 TW
Basically their reactor would vaporize if they tried to use pB11 with those numbers.
If I’m supposed to multiply by 1/2 in the power calculation, the efficiency and waste heat are even worse. Their input power (Pin) is also in the TWs. Good luck with that.
And notice how tiny their fuel flow rate is (due to the high Isp). That means low heat capacity in the fuel, which means high temperature, which means a lot of heat radiation hitting the reactor walls.
The biggest modern power plants are “only” a few GW. Those are BIG plants, with huge cooling towers etc. IF they can reach TWs, it’d be a game changer on many levels. But it’s a big if. Even way below that, waste heat is a big issue in space. If they’re claiming 3 TW, and using “only” 1 TW for thrust, good luck dissipating 2 TW of waste heat.
One way around that might be to place the reactor in a sort of thermos, with the walls reflecting most of the heat radiation back in. Run cold(er) fuel on the inside to carry away most of the heat, and turn it into more thrust (at the cost of Isp). That might help put most of the energy into the exhaust, leaving much less waste heat to dispose of by other means. But when thrust isn’t needed, they’d have to power down significantly.
They might be able to do something similar with the outer layers of their sheared flow, but they’ll have to raise their thrust power efficiency way above 30%. If we’re talking TWs, it’ll need to be at least 99.9%. That would get the remaining waste heat down to the GW level, which would still be very difficult to handle.
NewtonPulsifer raises another important point: if you try to squeeze that kind of power in a small package, you’ll end up running VERY hot (specific heat capacity is J/(kg*K); if there aren’t many kg, you’ll get plenty of Kelvins). If you don’t remove most of that heat before it reaches the walls, you’ll need unobtanium to handle those temperatures.
The X3 needs 102kW for 5.4N.
If you do the math for nuclear fission electric it is using 40% of the mass for radiators.
What about the radiators for this fusion one?
I can tell you didn’t do the math
That was a different group (General Fusion). Not related to them in any way.
Not true. E.g. IPA (UW/MSNW) produced neutrons from fusion.
I calculated the same and that was the one thing that I thought to be rather wild. They do seem to be assuming those levels of power though. In the table comparing He3 and PB11, they show a Pf (fusion power) of 3.3 x 10^12.
Either way, even with a fraction of the Isp, this would still be a game changer. In fact, I would rather trade Isp for more thrust anyway (maybe inject extra mass into the exhaust).
P ~= 1e5 N * 10 m/s^2 * 1e6 s = 1e12 W (1 TW). Not happening.
More likely they can select between either 1e6 s Isp (but low thrust) or 1e5 N (but low Isp), depending on how they run the engine.
Doesn’t matter what kind of nuclear you use. If you want high thrust and high specific impulse, you need a lot of power. Even with a modest Isp of 1000 s and modest thrust of 10 kN, you’d need:
P = F * g0 * Isp ~= 10000 N * 10 m/s^2 * 1000 s = 100 MW.
(give or take a factor of 2)
100 MW is a pretty big plant. But ideally you’d want at least 10 times as much Isp, and at least 10 times as much thrust, which would require 10 GW or more.
Ah, much better. “Specific impulses in the range of 10^6s and thrust levels of 10^5N are possible”.
Originally they were collapsing a lithium liner around the plasmoid and that was meant to absorb neutrons.
Maybe the story changed.
This one may be the closest thing imaginable to an Epstein drive:
https://www.researchgate.net/publication/239590484_Advanced_Space_Propulsion_Based_on_the_Flow-Stabilized_Z-Pinch_Fusion_Concept
While I like the DFD and the team behind it. There is another fusion space drive concept that could have much higher Isp and thrust. That is the Sheared Flow Stabilized Z- Pinch by Uri Shumlak. If I interpret the paper on it correctly, this one could potentially have a thrust to weight ratio above 1.
https://www.researchgate.net/publication/239590484_Advanced_Space_Propulsion_Based_on_the_Flow-Stabilized_Z-Pinch_Fusion_Concept
This is not a hobby reactor! What are you talking about? You are clearly trolling now.
Depends on the funding, mostly. We have made much quicker progress on much larger projects in the past. In any case, we need to fund projects like these much better. There are many out there. Many might fail but even if only a few of them succeed, the benefits would much outweigh the risks.
Because making it on Earth via D+D fusion is most likely cheaper.
Thank you for your reply. I concur with all that you are saying, with the addition that being 10 orders of magnitude from brake even means that this technology is really far from realization. It’s a science project in it’s early stages…..
Look no furthern than Nuclear Salt Water Rocket for that.
Just do NOT, I repeat, DO NOT point the exhaust at Earth.
Some of us can appreciate fusion (or fission) schemes from both a energy production and a propulsion point of view.
I think talking about the energy production point of view has little value aside from “which will break even first?”, because almost all fusion schemes will be much better than what we currently have.
“But fission is not that bad as people may it seem”.
I agree. But if most of the population believes it is THAT bad, it’s already a huge problem and cost to change or to deal with such perceptions.
So, getting back to the propulsion side of things.
Do they have papers with other mission profiles? To Mars? To Jupiter? Crewed?
What happens if you use 4, 10 of these together in a ship?
“This simple analysis indicated that assuming a fairly conservative specific power of 0.7 kW/kg, a mission was possible under 8000 kg using two 1 MW engines. A single SpaceX vehicle or Delta Heavy could launch the mission.”
Delta Heavy? If/when DFD is flight ready, Starship will be used to take this to orbit. Considering it’s diameter of 1,5 meters, and Starship 9m fairing, with some space to spare, you can probably put 13 or 17 of these into space, based on size alone.
If I go by what Dr Zubrin says of VASIMR, then it’s power supply needs to have impossible weight to power parameters.
“He3 is very expensive.”
Realistically, chemical propulsion with Starship will have already made near distance travel feasible and cheaper BEFORE fusion propulsion schemes are flight ready.
This means that Moon bases doing in situ chemical fuel production, doing astronomy, doing mining (Atkins Basin article) will probably already be in place. Primitive, but in place, to some capacity at least.
And if you have some small bases doing some little mining and fuel production, why not also harvesting He3?
Could you get some 10 of these together for a crewed spaceship?
How fast could it get 50 tons to Mars or to some Europa? (yeah, screw that Monolith)
I’ve been following this project for a while. Though it’s a very interesting concept, which I hope they’ll master, it’s still theoretical, as experts say it’s possible, however so far nobody achieved fusion in field-reversed configuration devices, so will see.
I’d like to add that Fusion power needs too much power up front, too much energy input, to ever work for humanity. The energy balance is there right in front of us; its very obvious.. The Sun doesn’t put that energy in to sustain itself, instead the Sun is like a form of degenerate matter where fusion balances gravitational collapse. The magnetic fields come forth from the sun, not the other way around where the magnetic fields contain the Sun. Anything but inertial confinement is just plasma physics, which has value in its own right. I get tired of hearing about multi tesla cryogenic space magnets that can operate with high capacity factor for 10 years on the way to Pluto – aneutronic or not.
The government has done quite a bit of research on its own; less obvious nowadays because we have peaked as a nation of purpose. Government operated a rather standard PWR as a light water thorium breeder reactor 45 years ago; it was a success but they dropped it. The government makes the specs, and what we call “private industries” or the “MIC” fulfil them. If we were close to making a torch fusion reactor based on the “Pretentious Princeton Pinch” configuration, propulsion would seem like a nice application for it. As matter of fact if we were close on any Fusion reactor besides an h-bomb, I imagine we could vent it and create thrust. this hobby reactor is another example of if we had some ham, then we could have ham and eggs, if we had some eggs. That’s a quote I heard on NBF last week.
Since we’re talking at least a few tons for a (small) ship, the thrust needs to be at least in the (tens of) kN range for those kind of accelerations. But the problem is that power is proportional to thrust * Isp, so if you want to keep the same Isp, you’d need to kick up the power by a few orders of magnitude – while still keeping it in a small enough package. Just the waste heat would be a big problem.
So for the foreseeable future, we have little choice but to take it slow, and use a rotating module for artificial gravity if we want to send humans (or tie two ships with a long tether and spin them up).
About 10 orders of magnitude, I would say.
While that is a good measure for how far along a fusion project is, it is not necessarily always a measure for the feasibility of a fusion approach.
There are other ways to look at it:
I see. So not exactly the Atomic acceleration featured in the TV show The Expanse, then. Well, that was 200 years in the future…
Their stated thrust is in the single newtons range. The proposed mission is on the order of 10000 kg. So roughly, 10 N / 10000 kg = 0.001 m/s^2. You need 9.8 N/kg for 1 gee.
Jumping ahead to manned flights to Pluto, would the acceleration of the spaceship be more or less than 1G? Well, that is actually somewhat optional actually, if one thinks of the acceleration – which is equivalent to gravity, says Einstein’s famous elevator thought experiment – as only one phase of a 3-phase acceleration/stasis/deceleration flight. In the acceleration phase one has to accelerate at 980.665 centimeters per second per second (https://www.merriam-webster.com/dictionary/acceleration%20of%20gravity)
in order to get 1G of force. Then one would need to have a stasis period of no acceleration, hopefully not too long since the astronauts would be weightless then, unless they moved into a set of rotating rings using centrifugal force to mimic 1G for that phase, then into a reverse (or reverseD) floor-to-ceiling section of the ship for the deceleration phase when nearing Pluto. Just staying within the rotating ring section would result in being thrown against the wall unless you were strapped in, which one could be I suppose, but for how long would that be acceptable?
The Apollo astronauts experienced short acceleration of multiple Gs, of course, but they only had to go a quarter of a million miles to the Moon, not 2.66-4.67 billion miles from Earth to Pluto (depending on where each is in their relative orbits). Nowhere in the article do I see where Pluto is relative to the Earth, but I assume the plan is to fly when the two bodies are in a reasonably close config.
@Skipjack
You seem to be in the know. How many order of magnitude is their reactor from brake even now? 10? 5? 20?
Thank you. That was the first time I’ve ever had a good reason why some fusion project would be wasting their time drawing spaceships.
Fusion funding below “fusion never” levels:
It is not BS.
For one, the government does not work that way. Congress cares more about jobs for their district that real progress.
Second, fusion funding is way too low. It is below the 70ies joke level of “fusion never”.
On top of that the government is putting most of the funding into Tokamaks and NIF. Very little is going towards alternative concepts, even if they are promising.
That said, Sam managed to publish some results with his reactor thanks to the funding from NIAC. Those results were encouraging enough that he now has somewhat solid funding from ARPA-E, which will help him take his machine further towards proof that it works.
VASIMIR needs a powersupply on top of the engine mass.
The DFD is both in one device.
For one that is not how the government works.
Funding decisions are political in nature. Congress cares more about jobs in their districts and the influence of lobbyists than progress.
Second, funding for fusion is too low, way too low.
There was a joke in the 70ies for fusion funding levels that was called “fusion never”. Actual fusion funding is way below that.
Without any reasonable funding, new ideas like this are hard to research and get published.
That said, Sam managed to get some funding from ARPA-E now (after they finally got details published with the NIAC grant). It is not much by any means, but it will allow him to further proof his concept.
Just saying it’s make believe. If there was something here, in this concept, the government would be funding the hell out of it and there would be a huge team of engineers. Takes a lot of skill to secure funding for such a piece of BS.
Sure, because nothing should ever be done for the very first time…
Not really much better,no. For an unmanned mission whether your isp is 5000 or 12000 doesn’t matter that much.
The potential advantage is the high electrical power it can provide on top of that isp.
EDIT: the radiators needed to bleed off megawatts of waste heat are also a serious engineering downside
D+D fusion is a lot more benign than D+T fusion.
So the bottom line is that He3 can’t really be a primary reactor fuel; You have to be running some other sort of reactor that produces oodles of neutrons, in order to end up with any significant amount of it.
Most of the power output is going to come out of THOSE reactors, not the He3 reactors.
Maybe in an economy that was using fission or D-T fusion as a major power source, you’d be getting enough He3 as a byproduct to justify using it in niche applications, such as, yes, space propulsion.
Much better than VASIMR, isn’t it?
I know the people behind this and they are serious and really good at what they do. Unfortunately, Sam’s lab is treated a bit like a stepchild by PPPL (which puts most of it’s eggs into the Tokamak basket).
Sam was alone most of the time, except for the occasional student, who got lost to his lab and barely had enough funding to keep the lights on.
They designed the Pluto mission for a NIAC grant. It is easier to get money for mission design than it is for development of a fusion reactor.
That said, Sam’s reactor DOES make for great space drive because of the way the scrape off layer transports off fusion products and out the back and because you can inject more mass into it to increase the thrust. Most fusion drives have very little thrust otherwise (but a huge Isp). This one has Newtons of thrust and still a great Isp of ~12,000.
Also worth noting that a confinement time of 300 ms is an impressive record for FRCs. TAE with their hundreds of millions of funding only got 12 ms so far. Of course confinement time is only one parameter, but it is still worth noting.
Anyway, the NIAC grants allowed them to publish a few papers on the reactor, which in turn finally landed them a significant grant from ARPA-E and that one will allow them to get development going a bit faster now. I am super happy for them.
They designed a mission for the space drive for their NASA NIAC grant. Paradoxically, it is easier to get money from NIAC for a pluto mission design using an in development fusion reactor than it is to get money for the development of said fusion reactor.
I have said that before too, when I talked to Stephanie and Sam. He3 is very expensive.
Now, there is one saving element that could still make this affordable:
You can make He3 with the same kind of reactor, if you burn D+D. Then one product is He3, the other is T, which decays into He3 within 12 years on average.
IIRC, the main reason why they are going with D+He3 is because of neutron wall loads and neutron damage to the reactor from D+T. The reactor is very compact, so neutron loads per m2 of wall would be pretty severe if it operated on D+T.
The guys did simulations for their NASA NIAC grants and yes, they are assuming a 4 year trip.
http://www.psatellite.com/tag/dfd/
“(why care, if no one is around to be irradiated?”
Well, EM radiation is easy to deal with, and charged particles are contained by the magnetic containment, but neutrons go right through that, so a significant neutron flux does complicate things considerably; First wall design, protecting superconducting coils from accumulated neutron damage, lost energy that you can’t use for propulsion.
I think it’s fair to say that all else being equal, (It isn’t, of course!) you’d rather have an aneutronic reaction, if only for the lifespan of the equipment.
Don’t believe the hype. Wake me up when its actually fusing. I love how they jump to speculating how fast they will reach Pluto. Get it working then you don’t need to speculate. BTW, don’t expect to attain the power of the gods without a supreme effort.
Well, clearly the article’s author(s) were repurposing their marketing position papers for spaceflight mode, without rethinking entirely the dynamics of “what makes sense” for the whole cost-energy-performance path.
For instance, quite a few words and paragraphs are keen to point out that the use of ³He + ²D is almost completely aneutronic (why care, if no one is around to be irradiated?), and moreover they have clever mechanisms to deplete the reaction chamber of ³T as it — as a fusion product — builds up. Again … why?
Indeed, your point is VERY well made: that if it is for fusion-to-the-outer-planets, then the “solution” need not have ³He at all. ³H + ²H is just fine. Higher fusion energy, useful daughter products, and all those sweet neutrons to alpha-activate some other transuranic, its alpha output being quite useful for propulsion in its own right.
⊕1 of course
Just saying,
GoatGuy ✓
I’m as much concerned about their running it on He3, and even Helium rich. He3 is freaking EXPENSIVE. It would be cheaper to feed it gold bars as reaction mass, if that would work. (Wouldn’t, of course…)
According to Wikipedia, US production of He3 was about 8Kg a year back in 2008. Sure, it could be scaled up, even enormously enough for this mission, but the price is never going to be good, and it would likely be decades before you could accumulate that much.
I wonder how hard it would be to run this thing off simple DT fuel, and just accept that it would be radioactive at the end of the burn?
The numbers… work out, except for transit time to Pluto (which isn’t given above). A “simple Lambert” flight path is modeled as “accelerate … D⋅⋅⋅R⋅⋅⋅I⋅⋅⋅F⋅⋅⋅T … decelerate”
One key ‘thing to overcome’ is the gravitational potental well of Sol. At EARTH, the escape velocity of the solar system (i.e. Sol at 149.5×10⁶ km ≡ 1 AU) is about 42,100 m/s. At PLUTO it is 6,650 m/s, for a ΔVesc = 35,440 m/s. That is the extra velocity needed by a projectile at Earth, heading out without other power, to end up at Pluto, having no further outward velocity relative to the lil’ demoted planet.
If 70% of the reaction mass (of (8,000 kg – 1,000 kg) = 7,000 kg × 0.70 → 4900 kg) is dedicated to outbound acceleration, with Isp of 12,250 (120,200 m/s effective thrust), having 2 MW input power, and all that, it is still about a 3.2 year trip. ⅔ of a year to accelerate, ½ year to decelerate, and 1.8 years of drift.
Some planetary billards (i.e. gravitation sling-shot assists) might be useful, but they do also take time to execute. The expected 102,000 m/s outbound (relative to Earth, as “instant start” ΔV) is high enough that it mightn’t be worth the time-and-course-distance investment.
Thing is … we’re also kind of counting our chickens before the eggs have hatched. Is the company actually demonstrating a single 1 MW thruster, or is this all theoretical?
Also, I find the 8,000 kg TKO mass almost laughably low. But who am I to say?
Just saying,
GoatGuy ✓
First of all, being first “aneutronic” reactor to mention side reactions in one of these press releases, let alone actual address how to minimise them, is a HUGE first. Kudos.
However this does not make up for the fundamental silliness of spending time coming up with all sorts of fancy space applications for a working fusion reactor years, or decades, before anyone actually gets one to work. Like designing an intercontinental train system before inventing the steam engine.
Actually, I don’t have to make up an example, here are multiple aircraft designs from the 1920s relying on the ability to beam electric power via a searchlight. (Spoiler: They never got the searchlight power beam to work.)