If SpaceX gets a fully reusable Super Heavy Starship flying to orbit in 2020 and then has 100 fully reusable flights by 2023 then the cost of space will drop by 100 times. This will start fulfilling the plans for lunar bases, lunar mining, and space-based solar power.
If each Super Heavy Starship costs $300 million and has $1 million in operating and maintenance cost per flight then the per flight cost is $4 million. Super Heavy Starship is supposed to launch about 100 tons to orbit.
Assuming that 800 Starlink satellites are launched by April 2020, then SpaceX will start doubling its revenue from $2-3 billion to $5-6 billion in 2020 and ten billion in 2021. This will mean that SpaceX will be able to afford to build dozens of Super Heavy Starships.
It will be trivial to create a lunar mining operation with a 100-ton fully reusable SpaceX super heavy starship. This will further reduce fuel costs by 5 to 10 times for orbits beyond low earth orbit.
Single-use SpaceX rockets have costs in the $1500 to 2000 per kilogram range. The reuse of first stages can bring SpaceX costs to about half their current costs. However, SpaceX will not drop prices that much without higher volumes and more price competition.
The lower costs will make it easy to develop significant space based solar power.
Laser light communication in a vacuum is physically 45% faster than communication through a fiber.
SpaceX will start generating substantial revenue in 2020 equal or slightly exceeding launch revenue. This was based upon 2017 SpaceX revenue projections from a 2017 Wall Street Journal article.
More photos from last night’s Starlink launch → https://t.co/095WHX44BX pic.twitter.com/u9rnacEz6O
— SpaceX (@SpaceX) May 24, 2019
Falcon 9 launches 60 Starlink satellites to orbit – targeting up to 6 Starlink launches this year and will accelerate our cadence next year to put ~720 satellites in orbit for continuous coverage of most populated areas on Earth pic.twitter.com/HF8bCI4JQD
— SpaceX (@SpaceX) May 24, 2019
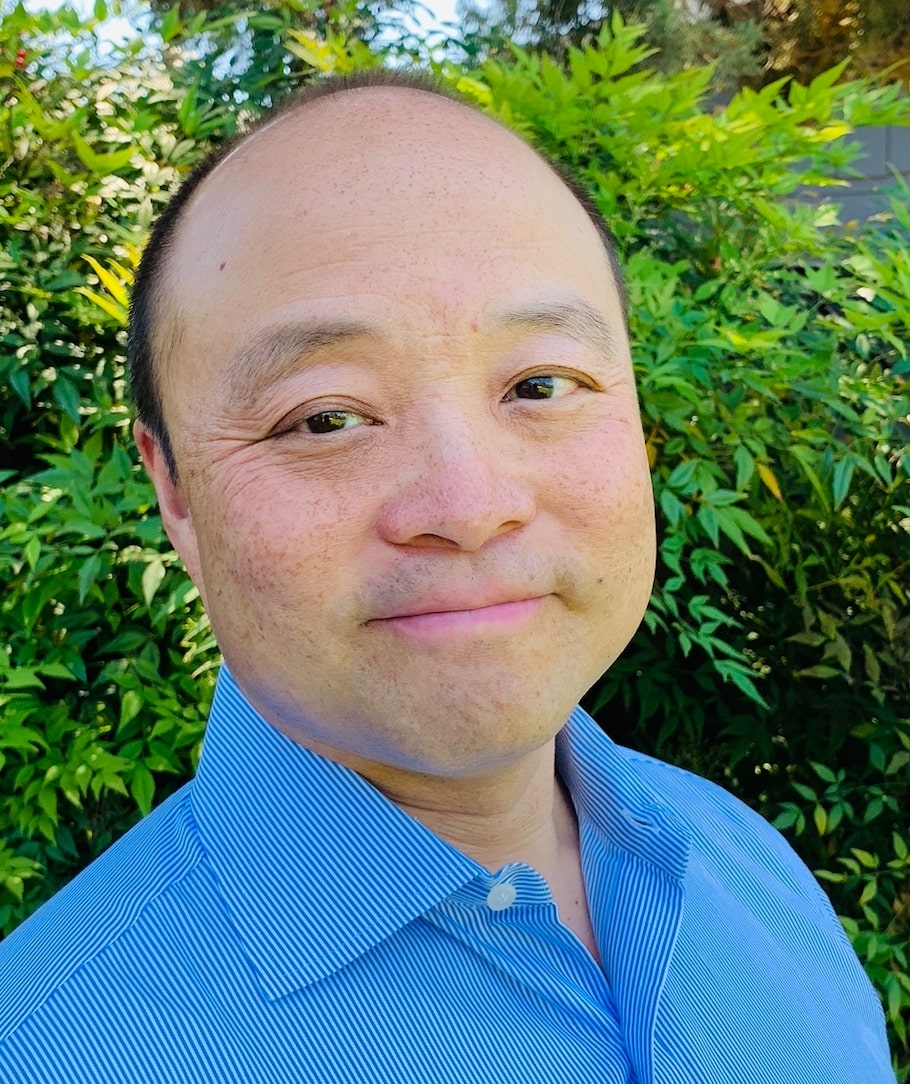
Brian Wang is a Futurist Thought Leader and a popular Science blogger with 1 million readers per month. His blog Nextbigfuture.com is ranked #1 Science News Blog. It covers many disruptive technology and trends including Space, Robotics, Artificial Intelligence, Medicine, Anti-aging Biotechnology, and Nanotechnology.
Known for identifying cutting edge technologies, he is currently a Co-Founder of a startup and fundraiser for high potential early-stage companies. He is the Head of Research for Allocations for deep technology investments and an Angel Investor at Space Angels.
A frequent speaker at corporations, he has been a TEDx speaker, a Singularity University speaker and guest at numerous interviews for radio and podcasts. He is open to public speaking and advising engagements.
Yes, I would call it the Eagle transporter.
However, the construction of SRBs is well understood. More closely related, hybrid propellant rockets have also been done (though admittedly they’re less developed). The latter use polymers as fuel, which is chemically similar. (Note that you’re not actually trying to build a rocket here – just a burner with a similar structure.)
Graphite itself is also well understood, and used extensively in industry. Graphite (and graphite composite) structures aren’t a new thing. And of course, chemical reactors and burners aren’t new either.
So even though this particular combination hasn’t been thoroughly analyzed, it’s really not that far outside of current state of the art.
The definition of TRL 6 is “System/subsystem model or prototype demonstration in a relevant environment (ground or space).” That holds true for pretty much all the subsystems here.
—
You are correct that there are hydrolox engines both developed and under development, but an engine does not a vehicle make. That said, by the time there’s any hydrolox infrastructure to speak of, there are likely to be vehicles too. I’ll admit that much.
There’s no such thing as a graphite SRB. I don’t have a clue what you’d use as a binder. I doubt very much anybody’s even done a serious analysis of what would be required–hence, TRL<2.
Note that Blue Origin will soon have three hydrolox engines in its stable: The BE-3, BE-3U, and BE-7. LH2 is a pain to handle and store, but you store it as water before refueling, and at the kind of scale and flight duration you need before boiloff becomes a big problem, you likely have enough solar power to cryo-cool your end-of-mission prop.
Applying the SOH Gas Implosion Engine, the spaceship’s launch cost will drop under $100,000 per launch.
Implosion Engines will provide 4-in1 : Propulsion, Power, Heat, and Electromagnet Shield against Radiation, consuming only reusable water, wasting not a single water molecule into the space.
Also, applying the SOH Gas Plasma-Flame Basalt-Rock Mining in natural undrground Lunar Caves, will offer a cost similar to the regular mining on Earth.
Basalt-Fiber Composite (BFC) Assembly-Modular Elements (https://basalt.alle.bg) for building Spaceships, Stations, Mining Tunnels Elements, Pipelines, Ship-Launch Silos, Machinery, Settlements, etc. Gigantic structures on the Moon and in the Space, will be the main industrial branch of the Lunar Economy. https://spaceship.alle.bg , https://asteroid-mining.alle.bg
Afterthought: If we go with the burner + Sabatier design, I’d say this is well past TRL 2. The carbon container/burner would basically be a solid rocket booster using graphite as fuel, except probably burning at a lower pressure than an actual SRB.
You leave a cylindrical hole in the center, same as with a regular SRB, feed oxygen into that hole from one end, and collect the exhaust from the other end. Feed the exhaust to a Sabatier reactor, split the water byproduct, and feed some of the oxygen and hydrogen back to the inputs.
SRBs are a mature technology, and Sabatier and water splitting are operational on the ISS as part of the life support system. Which leaves mostly integration work, placing this around TRL 6 or so.
It may be more expensive than pure hydrolox in the long run, but I still expect it’d be faster and cheaper to get this operational than a full hydrolox vehicle.
Let me know when that tech reaches TRL 2. And even then, it’s still way, way more expensive than just going with hydrolox.
Methalox makes all kinds of sense as both an Earth-launch propellant and as the thing to use for all martian operations. It just doesn’t work very well for the Moon.
I’m fine with using Starship as-is for as long as that set of hydrolox vehicles take to develop. But if SpaceX insists on sticking to methalox, they’re gonna get their lunch eaten, most likely by BO, who are perfectly happy developing hydrolox systems.
My methodology pretty much followed what you’re suggesting: I normalized Starship to a hydrolox-burning system by figuring out how much the current tankage should weigh, subtracting that from 90 tonnes (my guess what its dry mass will really be), then adding in how much hydrolox tankage would weigh to take its place. I got about 97 tonnes. If you want to go with the official 85 tonnes, it’d be 92 tonnes. Seems eminently doable.
As for lunar tankers/ferries, I figured out what you could fit into 750 m^3 (Starship volume with a little windage, so it’s easy to launch from Earth), and worked the mass of water to various orbits from that. I worked both all hydrolox made on the lunar surface and then transported, as well as just transporting water and making hydrolox in orbit (except for that needed to launch from the lunar surface, of course). Water was a clear winner.
As I said, you can make anything work with enough power. But what you’re describing is, for at least the next 20 years, science fiction–especially when it comes to moving even tiny NEAs.
I’m interested in booting up a water operation that can reduce the cost of lots of cis-lunar operations and the occasional Mars mission in the <15 year timeframe. You need that before you have the ability to put rubble processing operations anywhere.
I’m more interested in the next 15 years, not the next 50. TRL 2 technology is OK; TRL 0 probably isn’t.
Since asteroids are all in motion relative to Earth, you don’t process them in-situ. Rather you haul the raw rock back to where the customers are, which is mainly around Earth for now. Your processing equipment stays put, and the mining tug visits a different asteroid each time, whichever is aligned best at the time.
Hydrated minerals have bound H or OH groups in their formula. When you heat them, these groups dissociate, leaving a different mineral as residue. The carbon compounds are a tarry substance similar to kerogen, the precursor to petroleum. When you heat that, the light molecules vaporize, and the heavy molecules break down to lighter ones. This is similar to cracking towers in refineries.
How fast all this happens is a function of particle size and surface area. People doing oil shale extraction in-situ (underground) would know whats required,
Plasma engines function differently than ion engines. You beam microwaves at your fuel, tuned like a microwave oven is to the right frequency. This heats it enough to become a plasma. Then an RF heater further heats the plasma to ~1 million degrees. The technology to contain the plasma is borrowed from fusion research. The plasma escapes through a magnetic nozzle. Everything becomes a plasma when it is hot enough, so you can make it work with any fuel.
Regarding carbon handling (ran out of room), yes, I’m thinking ground graphite or something similar. Not sure how finely ground – small granules may work well enough. You can play with the porosity to get the surface area where you want it.
On Earth, powder or granules are usually loaded into hoppers, and fed from them to wherever they’re needed. So you could have a hopper-shaped container. But depending on the process details, it might be as simple as a sufficiently strong insulated tank made of appropriate materials, with an inlet for hydrogen and an outlet for methane + unreacted hydrogen. You’d only pump the gases in that case.
Another option is wash it with liquid water. Then you’re pumping a slurry. If that slurry is heated high enough, the water would oxidize the carbon, similar to water gas shift reaction. This would produce what’s known in industry as “water gas”, which can be passed to a Sabatier reactor.
The problem with using hydrolox directly, is you need dedicated vehicles for that. So there are basically 3 options:
1) Use the existing (methalox) vehicles, and carry all of the methalox with you (including LOX).
2) Build hydrolox infrastructure + design, test, and build new hydrolox vehicles to use it.
3) Build hydrolox infrastructure + add the necessary bits to convert it to methalox + use the existing vehicles, but carry only the carbon.
You may be right that the economics of hauling carbon are bad (I can’t easily comment on that), but surely they’re better than hauling all of the methalox, if we neglect the cost of the extra infrastructure for a moment.
So if you’re going to invest in the hydrolox infrastructure anyway, then the comparison reduces to adding the bits to convert it to methalox (and then using the existing vehicles) vs designing and building new vehicles to run on hydrolox.
As I’ve noted in my previous comment, the extra bits for making methalox are relatively simple, so I imagine both their cost and their time-to-market should be far lower than that of new vehicles. So in the short to medium term, there may still be some merit to it, even if it compares poorly to hydrolox-only in the longer run. But in the longer run, I agree that dedicated hydrolox vehicles would be better.
A huge part is missing in these calculations.
If it only costs 4M to send 100+ tonnes into LEO, then pushing for lunar fuel really is almost a waste. It will cost plenty to get mining and launch equipment up there. In the mean time, costs will only keep going down here.
If you’re visiting a rubble pile, you might just want to use O’Neil’s “slinger” engine. It just feeds sand or dust out to the end of a rotating arm, and lets it go at the right moment.
ISP was expected to be terrible, but the reaction mass would be plentiful.
If the O:F mixture for Raptor methalox is 3.58, then carbon is about 16% of the total mass of the prop. I get about $300/kg cost for 150 tonnes of regular Starship mass to the lunar surface, if you assume that launches are $5M (it takes 1 payload + 8 refueling launches to land on the lunar surface). So you need 160*300 = $48,000 of carbon per tonne of propellant.
In contrast, excluding capital equipment amortization, I get about $700/t to harvest water and convert it to hydrolox on the lunar surface. So the economics for carbon transport are… not great, to say the least. I’m willing to believe that I’m off by a factor of 10 on lunar hydrolox production costs. It’s still not great. Even if I’m off by a factor of 100, you’re still almost doubling the cost of the product.
One other problem: How do you plan to transport “carbon”? As ground graphite? Any thoughts on how to move it around? Do you pump it? Do such pumps exist? Do they work in 1/6 g? For that matter, if you want to manufacture prop in orbit, do they work in microgravity?
I’ve mentioned before: if you’re making hydrolox from Lunar water, it’s not very hard to convert it to methalox using carbon from Earth. That still gives significant mass savings over hauling all of the fuel from Earth (or even hauling just the methane).
You could possibly pass hot hydrogen right through the carbon powder, and get methane out the other end. Then pass the methane through the same liquefaction equipment you’d use for the hydrolox. Or more conventionally, react the carbon with the oxygen, then pass the result through Sabatier. Split the water byproduct and repeat.
Compared to making hydrolox, the former adds only a carbon hydrogenation reactor (and maybe a centrifuge for separating methane from unreacted hydrogen), the latter adds a burner and a Sabatier reactor. Neither is particularly complex. NASA has a compact Sabatier reactor on the ISS.
This may not be the ideal solution, but it may still have cap-ex and time-to-market advantages over developing several additional spacecraft to take full advantage of hydrolox.
(Note: To minimize delta-v costs, you’d probably want to bring the carbon and water to where the methalox is needed, and make the methalox there.)
(Note 2: You could probably skip the centrifuge, since methane and hydrogen boiling points are 90K apart. You should get decent separation during the liquefaction process.)
By “dig up”, I mean that you have to move regolith and rocks through an oven. I don’t think you can just vaporize it in situ, as several plans propose for water ice. That makes everything a lot more complicated.
As for plasma rockets, low ionization energy is a big deal. None of CH4, H2O, or O2 are easy to ionize. That’s why most electric propulsion uses some kind of noble gas. If you want to carry around a bunch of extra dry mass for more power, you can make almost anything work, but your solution doesn’t necessarily close.
I’m well aware of what form the water is in. You need kitchen oven temperatures (200-300C) to bake the water and carbon compounds out. This is not hard because at 1 AU, the black body temperature in full sunlight is 120C. Moderate solar concentration will do it.
Not sure what you mean about “dig up”. Look up photos of Ryugu and Bennu, the two asteroids being visited by probes. They are “rubble piles”, with lots of surface boulders. You grab a suitable sized one and go home.
Plasma engines are not picky about propellant, everything is a plasma at a million degrees. Oxygen and methane work just fine, and are reasonably storable. So does water. You need a load of propellant for the first trip, but it is self-fueling after that. You produce up to 20% volatiles from the asteroid rock, and you need 2.6% to fuel the next trip. Anything above that can be used for other purposes. The other 80% of the asteroid rock can be further processed for other things, but propellant is the first necessary product to make it all work.
I think he should begin to annex his laboratories…holistic monuments.
What about a mountain top observatory? (Shush)
What do you think? 1:50PM 06/12/2019
http://www.rexresearch.com/testatik/testart.htm#csmith
magnetic quantum key diaphorum
https://news.ncsu.edu/2019/06/lsmo-sandwich-structure/
https://van.physics.illinois.edu/qa/listing.php?id=1256
The Cosmic Ether: Introduction to Subquantum Kinetics
Paul A. LaViolette
http://www.antigravitytechnology.net/thomas_townsend_brown.html
A Student’s Guide to Maxwell’s Equations by Daniel Fleisch
https://www.theverge.com/2019/5/22/18634401/huawei-ban-trump-case-infrastructure-fears-google-microsoft-arm-security
https://www.youtube.com/watch?v=1u5pNNnFflg
16:02
3+1 click
http://hermital.org/book/holopara1-1.htm
Nassikas Reactionless Lorentz Thruster Project – Dr. Paul LaViolette
Yes, I am missing an E8.
I have a model that looks at Starship-sized logistics with lunar prop available at LEO, LEO+2500, NRHO, and the Moon. I’m using two hypothetical spacecraft here:
a) A 750 m^3 lunar tanker, which can have a 150 tonne water tank stacked on top of it. (This should allow the tanker to be delivered to LEO in one Starship launch.)
b) A hydrolox version of Starship, i.e., one that delivers 150 tonnes to LEO, but has larger (heavier) tanks to accommodate the LH2.
Here’s what I get when I compare the hydrolox-sized Starship, using lunar prop provided at LEO, HEEO (LEO+2500), NRHO, and the lunar surface, to the current Starship design, using methalox delivered from Earth:
1) For 150-tonne payloads to Mars, refueling once in LEO and once in HEEO (which is just about halfway, delta-v-wise), the prop costs about 30% compared to the Earth-delivered methalox option.
2) 150 tonnes direct from LEO to Mars, prop costs about 50% using the lunar hydrolox than the Earth-delivered methalox.
3) 150 tonnes LEO-to-NRHO-to-lunar surface, and then back the same way empty, prop costs about 15% of using Earth-delivered methalox.
But we’re not considering the capital costs yet. These numbers will get worse. Lunar water will still almost certainly be a winner, but it’s not a 100x winner by any means.
If there’s a supply of volatile-rich rocks, then why do we have to worry about depleting the ice reserves? Doesn’t it make more sense to harvest the easy stuff first, so that the technology has improved by the time you need to mine the hard stuff?
Ladies and gentleman i present you a perfect chance to lose your weight. Do it hard enough and you will have your moon trip almost for free
I hope we have wizened up and developed a robust asteroid mining economy by then!
Or alternatively, mass drivers to launch lots of stuff into space without spending aby in situ water.
With a supply of volatile rich rocks and easy access to Moon materials, we can avoid depleting the Moon of water (it will need it for other things than fuel), and have much cheaper fuel and cargo in space.
The water in NEAs is locked up as hydrated compounds. To release it, you have to dig up the regolith and rocks and bake the water out of them. On the Moon, we’re pretty sure that there’s water ice, which can be volatilized in situ, making it a lot easier to harvest.
As for transporting stuff via electric propulsion, you’re gonna need a viable in situ propellant to make this worthwhile. Otherwise, launching and transporting the prop to the proper place pretty much eats up any savings you’d have.
I suppose that eventually we’ll have to worry about depleting the Moon’s water resources. But if this actually gets to be a problem, the cost of importing water to the Moon is likely to be low enough that it’s not an insurmountable issue. Meanwhile, for the short term (i.e., the next 15 years), lunar water seems like it’s the only game in town.
Agree. All these space habs and labs were made as public research projects, where the low cost really isn’t the parameter to optimize.
If they had to make tens or hundreds of habs, though, then economies of scale and mass production would come into play.
And no, Bigelow really isn’t an example of that, because their pricing is certainly cheaper than the ISS, but still very in line with the cost of other space projects, and they haven’t had the chance of launching one fully functional habitat yet.
They could get there, if they become capable to start putting modules in orbit, lease them or sell them to others and then require to add more.
And there could be others, SpaceX and Blue Origin included, that could start launching habitable modules of their own making. A modified Starship could perfectly be used as a permanent space station module. And I know BO wants to turn empty New Glenn second stages into habs too.
Some near Earth asteroids contain up to 20% water and carbon compounds. These can be reformed to oxygen and methane to feed your engine. An electric tug can haul 160 times it’s starting mass back to an orbit near the Moon, during a 15 year operating life (6 round trips). Therefore it can supply up to 32 times it’s mass in fuel, net of the propellant the tug itself needs.
With a depot near the Moon, you can travel up and down all you need. I would reserve the limited Lunar water supply for use on the Moon itself. Sure, for the first few flights building up a base, you can use local water for propellant, but other people have small LOX-LH2 engines already. Notably the RL-10 engine has been around since 1960, and Blue Origin has developed one for their lander.
The lowest cost option for Space Solar is using the solar farms already built on the ground, and aiming the extra photonic “light pollution” from Starlink at them during the 3 hour periods around Dawn and Dusk. You nearly double daily electrical output because Solar Panels are very inefficient at low light levels, and never have to exceed local noon light intensity. This is Dr. Lew Fraas’s concept. This adds billions to SpaceX’s revenue stream with no additional launches.
if anyone wants to watch a great movie that just came out addressing this latency issue please watch The Hummingbird Project… has a great explanation
That would explain why women never replied to my emails…
In the 1980s I attended a conference on the highly touted Space Plane – nothing ever came of it – and so to are these ridiculous claims.
It will be interesting when an economy ticket into orbit is cheaper than a first class ticket across the Pacific Ocean. I suspect Bigelow will start building orbiting hotels and space tourism will be a real thing for the middle class. Now, about all those other predictions… I’m not so sure…
If not these and first stages after economic life is over would be the cheapist space stations and planetary transit vehicles
I most hope that he is wrong as well or is proved mostly wrong
Speed of light in vacuum = 2.998E8 m/s.
Speed of light in fiber optic cable = 1.999 m/s.
Playing the game on different markets is exactly where the low latency trading comes in. It’s a simple arbitrage play.
You need to do the math on fiberoptics vs. satellite internet transit. Speed of light in fiberoptic cables is only about 65% of the speed in vacuum and air. Two 500 km hops up and down to reach the satellites costs considerably less than the cost of the slower fiber connections.
One other thing that I’ve been trying to model (with limited success): If you start a lunar water operation, you are first and foremost your own best customer. That means that even minor increases in third-party demand for prop can have big scaling effects on the operation, because they require more logistical flights, which in turn leverage the water operation. This kind of recursive use of your infrastructure to scale up is hard to model, but I’d guess that operational costs drop much faster than you’d think, as a result.
I really have to wonder if SpaceX would have chosen methalox if they’d known that NASA was going to concentrate on the Moon for a while. Methalox is absolutely the right choice if your two main targets are Earth orbit or Mars, but it is most decidedly not the right choice for cis-lunar transit, especially when that transit operation is going to be supplied with water-based prop. I wouldn’t be incredibly surprised to see them go after a low-to-moderate-thrust hydrolox engine for that purpose. Launching a transit tug and/or lunar lander that fit in the Starship cargo hold seems like something they’re going to need to do if cis-lunar is where the money’s going to be for a while.
Parts of the industry, mostly the manufacturers and integrators of actual spacecraft, will indeed need time to adjust to the “heavier and less reliable is better” environment that a 5-10x reduction in launch costs brings about. But there are three areas where big payloads can be deployed immediately:
1) Propellant or other bulk consumables. Prop is clearly the leading contender here, but another one that will be high on the list is a Big Bag o’ Solar Arrays.
2) Big tankage. It doesn’t take much time to fling dumb bulky stuff into LEO.
3) More megaconstellations, with Starlink obviously being the early bird here, but scaling the number of units of existing low-mass/high-reliability designs is a manufacturing problem that’s tractable pretty quickly.
#1 and #2 obviously scream “prop depots”, and I’d guess that that may easily be a biz that SpaceX wants to be in directly. There’s a big advantage to being able to leverage non-integral numbers of tanker launches, which requires storing the leftovers needed for the heavy BEO missions (or heavy GEO missions, for that matter).
OTOH, you can use separation processes that aren’t used on Earth, like electrostatic levitation. Maybe large scale mass spectroscopy. It’s nice that you can separate a fairly pure nickel-iron component that way.
What you’d have to stay away from are any processes requiring water or carbon.
Also, without an atmosphere to diffuse sunlight, parabolic mirrors can achieve some seriously high temperatures. Easy to bake off any volatiles, even melt lunar soil.
Direct satellite delivery of television to a small residential dish began service over twenty-five years ago. To subscribe, a person had to acquire and install their own ground station mini-dish, a set top convertor, connect the system to a wired phone service, and arrange a payment option before the era of the ubiquitous debit card.
Many people were fed up dealing with cable television pricing, it’s limited programming repertoire, and poor customer service. Many more had no option but the programming carried by a handful of broadcast networks. An equipment and installation cost initially about $300-$500 of early 90’s money did not deter; instead, great demand rapidly ensued once the word spread.
If one in the present circumstance desires internet with sufficient speed of delivery to support a 4K television program, allow a reasonably speedy connection to conduct a business operation’s file sharing, or support interactive video conference capability, the options for service may be limited or unavailable outside a metropolitan area.
The ISP if available often levies high charges for minimal service delivery, when contrasted with markets of no more than a half-hour travel to visit. Many potential clients live outside properly serviced areas altogether, with their only recourse the offerings of entrenched satellite providers offering low bandwidth, high latency connections. StarLink will present an attractive service option, relative to the non-responsive legacy system.
That is based on playing the retail game. I bet SpaceX could produce propellant on the earth and fly it to the moon for their own use at a better ongoing price point. The cost to itself will never be the same as the cost they charge their customers for the same service.
One aspect of Lunar mining is easier than on Earth. A long history of impacts has left a layer of broken rocks down to dust that is 2-8 meters thick across the whole Moon. So you don’t need to do blasting or crushing, it is already done for you.
One downside is Lunar dust is very abrasive, and tends to stick to things electrostatically. Another is impacts flinging stuff around makes for low grade ores – one part of the Moon is much like another, with a few exceptions.
It should not be surprising. When we (Boeing) were building the Space Station modules, we got our aluminum from the same place we got it for passenger airplanes.
More generally, aerospace companies build rockets in the same kinds of factories, with the same kind of engineers and technicians, and using the same kinds of materials as airplane production. The main difference is typically a lot fewer rockets are built, so the initial R&D is spread over fewer production units.
Very exciting times upcoming. It seems the market and tech are finally ready to bring some of the dreams of the early space age to life.
If a 2 orders of magnitude decrease in cost per pound to LEO comes to materialize in the next decade or two, many of us alive could expect to see orbital trips in their lifetime, becoming almost mundane occurrence. And richer folks could expect to do visits to even farther places, for sightseeing and business. I know I’d pay a few thousands for the chance of experimenting 0-g and doing some orbits around Earth in a Starship.
That would be a future many of us have long awaited for.
The hitch really is the development of commercial space facilities (which nowadays is difficult and expensive), increasing the launcher’s reliability and gradually grow a market based in production in series of items, instead of one-shot research products.
But I foresee that as more things can be launched, there will be a concomitant reduction in price on space worthy items and machinery.
“space-based solar power” such as http://www.searchanddiscovery.com/pdfz/documents/2009/70070criswell/ndx_criswell.pdf.html
I will refer to this in my answers!
What I would certainly anticipate are teams being gotten together to address the following question: “Here are five price points for cargo to LEO. What are the new products we could make money on at them, and what do we need to do to be first to market for them?”
“It will be trivial to create a lunar mining operation…” No. The only trivial thing in space is dying, the rest is hard.
I predict that you are wrong on these points and many other points.
Yep. If it can be reused a high enough amount of times, it can have similar economics and price per pound.
If not, it can have a higher cost, but still much better than anything we have had so far.
$300 million is around what a Boeing 777 costs. Think about that for a minute.
His point is that you can be co-located with the NY stock exchanges servers in Jersey, OR you can be co-located with servers in Japan, but you can’t be both at once.
The low latency permits you to get inside the loop for traders using slower connections for trades between different locations, even if it doesn’t help for local trades.
Mind, I tend to think the sort of trading that demands these low latencies isn’t really contributing anything to the real economy, and we’d likely be better off without it.
” Solar panels and space furnaces can generate enough energy to process their own mass of ore in a week, even for energy-intensive processes.”
Indeed, I once calculated that a Von Neumann machine would have a fairly decent doubling rate even if it had to construct everything by a process as energy expensive as molecular beam epitaxy.
Particularly since solar panels made IN space would not have to be constructed to survive the rigors of launch, or exposure to our atmosphere. They could be barely more than the active material, in zero g vacuum applications.
Having worked on space systems at Boeing, you can’t design a payload until you have the equivalent of the Falcon User’s Guide https://www.spacex.com/sites/spacex/files/falcon_users_guide.pdf
It may not be the published final document, but you need the equivalent set of information. So far as I know, SpaceX doesn’t have that information ready yet, and won’t until the second, high altitude Starship prototype has flown, and the SuperHeavy first stage has at least done a static engine firing.
Payloads going to space need to know the payload mass, dimensions, center of gravity allowances, vibration levels, g-forces, thermal conditions, pressurization (or lack thereof), and mounting interfaces (mechanical, electrical, data, and fluids, if any). Until they have all that, they don’t know what they are designing to withstand, and most of that info isn’t available until the vehicle design is finished and tested.
You can do preliminary work, like optimizing solar arrays, propulsion units, and structure for a lower cost to orbit, but not actual design of a payload.
I predict almost none of this happens. We are and will be earth-bound creatures for a long time. Moon bases will most likely be automated factories of some sort. Star link is not guaranteed. Who are all these customers without internet service? The ones without it usually don’t have $$ to pay for it. As for the stock market latency conversation. Latency issues affect traders, not investors. Investors are wealthier than traders. Ray Dalio vs. Buffet. Who has more money? Buffet doesn’t worry about latency.
The implication of your conclusions is Spacex’s [would be] future customers are not already redesigning their payload to fit the $200/kg costs and the bigger size Starship will allows.
I’m pretty sure many are already working on that and many more will follow.
In a related matter, if putting stuff in orbit is cheaper, design costs and times can/must be reduced considerably per single item (so a single unique item will be able to afford a lower cost of design or the cost of design MUST be divided by multiple items).
Entangled comunications will be be the definitive low latency solution.
… The other thing not accounted for in your post is that off-planet resources can supply 98-99% of the mass and 100% of the energy to build things in space. In the early days this will be much lower, starting with propellants and bulk shielding. But whatever percentage comes from off-planet leverages the mass you launch from Earth.
The 1-2% includes elements and compounds that are rare in space, and more easily supplied from Earth, and hard-to-make items already mass produced here, like computer chips. They are high value-to-mass items, so the cost to launch them is small. It is not worth setting up factories to make them in space for a long time.
Starship launch costs, even at the start, are low enough to bootstrap space mining and production. Solar panels and space furnaces can generate enough energy to process their own mass of ore in a week, even for energy-intensive processes. The rate-limiter will be the remaining process plant mass, and how fast individual process steps will take. Once started, such industry can rapidly grow, by making more equipment for itself.
The total mass launched to orbit in the history of spaceflight is on the order of 10,000 tons. Even the full Starlink constellation of 12,000 satellites is on the order of 2,700 tons, over about 6 years.
So I claim there isn’t 100 Starship flights per year (i.e. 10,000 tons) of payloads available to fly at present. 6-10 per year is a more reasonable number to start with. There’s a certain amount of fixed annual overhead to keep a Starship flying – launch site crew & maintenance, low-level engine production, flight operations (mission control), etc. That fixed overhead had to be divided into the annual flight rate. On top of that you have the variable costs, like fuel and vehicle life usage.
Assume that SpaceX needs 500 total people to keep the Starship flying, and their loaded cost is $200K/year (salary, benefits, office space or shop equipment). That gives you $100M a year, plus maintenance and hopefully a profit margin. Divided by 10 flights a year, that comes to $20 million/flight, which is what I think a realistic *starting point* is for Starship prices.
Spacex’s customers will respond to a price of $200/kg by designing payloads to be heavier and cheaper, and more than one-of-a-kind items like the Space Station. So traffic to space will increase, but not instantaneously. I assume about a 10 year response time for the industry to adjust to the new reality. As traffic goes up, the cost per flight will go down.
This is correct. For example, the New York Stock Exchange’s main data center is in New Jersey, where there is room for large, modern data centers. Brokerage houses co-locate their computers in the same data center. There isn’t much you can do to reduce latency when your rack is only 100 feet away from their rack.
The building on Wall Street is a tourist attraction these days. Anything that involves a human step, like hitting “enter”, adds more latency than being on the far side of the Earth.
You wrong. The trick is to use arbitrage between remotely spaced stock exchanges, to use minor price fluctuations between exchanges , so for example I buy shares MSFT at stock exchange A and millisecond later sell it on exchange B for a couple of pips profit, before market moves. In reality its more complicated
The rules are the same for everyone. The biggest players will always get the lowest latency and benefit, just the name of the game.
Low latency argument is no B.S. There are projects in the works right now to lay down an optic cable on the ocean floor specifically to reduce latency for high frequency traders and it is worth billions.
‘low latency’ argument is BS. Those who do this work are already physically very close to the stock markets they want to ‘play’. For a signal to hop from the ground to the nearest Starlink satellite then back down (to/from New York for example) is already much slower than the direct F/O links they have now. People in CA or anywhere else is the world cannot play this game on the NYSE, and beat those physically next door.
However, if playing this game between different markets, then it may help. Hopefully they’ll change the rules of the game to not allow profiteering by those with the biggest wallets (aka lowest latency).