Astronomers turned networks of dead stars into galaxy-size gravitational wave detectors, radio astronomers have tuned into the slowly undulating swells in spacetime thought to arise from pairs of supermassive black holes (SMBHs) that are about to collide.
We are improving instruments and creating new instruments to improve our gravitational map of the universe.
“As a group we’ve agreed to avoid the D-word,” says team member Scott Ransom of the National Radio Astronomy Observatory. This is a 3.5 to 4 sigma level confidence versus 5 sigma needed for a Discovery.
In a simultaneous announcement today, five separate international teams said that after nearly 20 years of effort they had found evidence for these gravitational waves. They are far longer than the waves first captured by ground-based detectors in 2015, which emanate from collisions of star-size objects. The findings not only open up a new window in gravitational wave astronomy, but will also help researchers answer questions about the origin and evolution of SMBHs, objects that sit at the center of galaxies and weigh as much as billions of Suns. The results could even uncover hints of new physics.
The new results rely on millisecond pulsars, highly magnetized stellar remnants that emit beams of radiation as they spin as fast as 1000 times per second. Their lighthouse-like radio signals sweep past our planet with a regularity that rivals an atomic clock. Should a passing gravitational wave intrude anywhere along the path between Earth and the pulsar, it will stretch and squash the fabric of space—and cause small variations in the timing of the flashes. “Those ultraprecise signals will arrive a little bit early, and then a little bit late,” says Chiara Mingarelli, an astrophysicist at Yale University and a member of the North American Nanohertz Observatory for Gravitational Waves. NANOGrav is one of the five international pulsar timing arrays (PTAs), which draw on data from a dozen radio telescopes around the world to monitor dozens of the beacons for evidence of the waves.
The challenge is immense because of the many potential corrupting sources of noise: radio interference from terrestrial technology and satellites, scattering of the pulsar’s radio beams by intervening clouds of gas, and tiny random variations in the spins of the pulsars themselves. “You have to beat down all these confusing signals,” Hessels says.
The fact that the PTAs detected a signal at all suggests that friction from gas and dust—or other stars and black holes—must be helping the SMBHs shed energy and pass the stall-out threshold.
The background signal is stronger than expected, implying that this population of imminently merging SMBHs numbers in the hundreds of thousands, perhaps even millions. It could also mean the SMBHs are either bigger or merging more quickly than astronomers previously predicted—a possibility consistent with observations from NASA’s JWST space telescope hinting that galaxies grew larger and faster in the early universe than once thought.
By mapping how the overall background varies on the sky, the PTAs might eventually get good enough to detect individual SMBH pairs in the nearby universe, enabling other telescopes to zoom in to study what happens in galaxies after they merge. More pulsars would help. The Canadian Hydrogen Intensity Mapping Experiment, a radio survey telescope, is on the hunt, and China’s Five-hundred-meter Aperture Spherical radio Telescope, the world’s biggest, is finding pulsars too faint for other facilities.
The European Space Agency’s Laser Interferometer Space Antenna (LISA), a space-based detector, might help fill out the spectrum of gravitational waves. LISA, which is scheduled to launch in 2037, would be able to see gravitational waves in a wavelength range between those observed by LIGO and the PTAs. These would come from mergers of intermediate-size black holes, those between 100,000 and 10,000,000 times our Sun’s mass, such as the one that lives in the center of the Milky Way.
But NANOGrav investigators now feel they have strong evidence for the waves, in a 15-year data set of 67 pulsars located up to 20,000 light-years away. They identified anomalies of one part in a quadrillion—comparable to measuring the distance between Earth and the Moon to within 1/1000th of 1 millimeter. The team is reporting the detection at a 3.5- to 4-sigma level, indicating more than 99% confidence that the signal is real. However, this falls short of the 5-sigma threshold that physicists often want before claiming a discovery.
The signal is very different from what ground-based detectors such as the Laser Interferometer Gravitational-wave Observatory (LIGO) pick up. Those are sensitive to the milliseconds-long blasts of waves produced by individual mergers of black holes and neutron stars with masses tens of times that of the Sun. The pulsar timing results instead represent many waves, each years to decades long, overlapping in a cumulative background hum. Each wave is generated by a pair of SMBHs somewhere in the universe whose host galaxies have collided and merged. The black holes orbit one another for tens of millions of years, emitting gravitational waves in the lead-up to an eventual merger. (Unlike LIGO, the PTAs are not sensitive to the mergers themselves—just the death spirals.)
The background signal could include other, more exotic sources. Primordial gravitational waves might have been generated shortly after the big bang if the universe experienced convulsions from a still-hypothetical breakneck expansion in size known as inflation. Some theorists also posit that the violent emergence of one of the fundamental forces at the dawn of time left defects in spacetime called cosmic strings, which would now stretch across the universe, vibrating like piano wires and emitting gravitational waves in the range that PTAs are sensitive to.
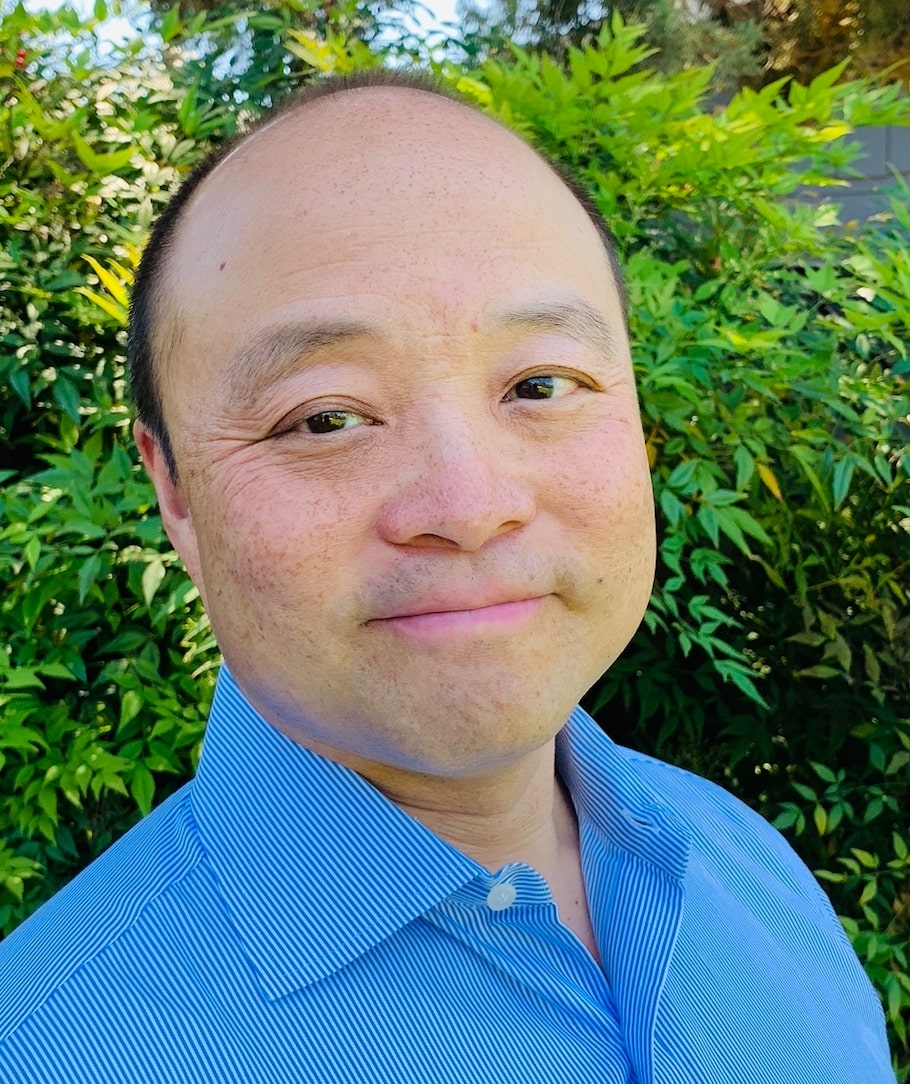
Brian Wang is a Futurist Thought Leader and a popular Science blogger with 1 million readers per month. His blog Nextbigfuture.com is ranked #1 Science News Blog. It covers many disruptive technology and trends including Space, Robotics, Artificial Intelligence, Medicine, Anti-aging Biotechnology, and Nanotechnology.
Known for identifying cutting edge technologies, he is currently a Co-Founder of a startup and fundraiser for high potential early-stage companies. He is the Head of Research for Allocations for deep technology investments and an Angel Investor at Space Angels.
A frequent speaker at corporations, he has been a TEDx speaker, a Singularity University speaker and guest at numerous interviews for radio and podcasts. He is open to public speaking and advising engagements.
Clever humans.
Very exciting, wish LISA would launch sooner.