Arxiv – On-chip quantum phonodynamics (6 pages)
ABSTRACT – Sound can be just as quantum as light. But our toolbox for single quanta of sound, i.e. phonons, is currently insufficient. Here we describe a new component that enables a chip-based, solid-state analogue of cavity-QED utilizing acoustic phonons instead of photons, phonitons instead of polaritons. We show how long-lived and tunable acceptor (hole) impurity states in silicon nanomechanical cavities can play the role of a matter non-linearity for coherent phonons just as, for example, the Josephson qubit plays in circuit-QED. This system enables the control of single phonons and phonon-phonon interactions, dispersive phonon readout of the acceptor qubit, and compatibility with other nano/optomechanical components such as phonon-photon translators. Phonons, due to their unique properties, enable new opportunities for quantum devices and physics.
(and of lowest acceptor states) corresponds to particles of spin J = 3=2.
(b) Ground state splitting via external magnetic field along [0;0;1] direction; allowed (forbidden) phonon transitions and qubit phonon driving (text). Level rearrangement is via additional strain. System manipulation via electric static/microwave fields is possible. (c) Examples of nanomechanical 1D and 2D phonon bandgap cavities reminiscent of already fabricated high-Q cavities in a patterned Si membrane. The acceptor is enclosed in the cavity and an on-chip phonon waveguide allows phonon coupling in/out of the system.
Technology Review has coverage
The new idea is the phononic equivalent of circuit-QED. So instead of trapping a photon in a cavity and making it interact with an artificial atom, these guys want to do it with phonons: quantum phonodynamics.
Their scheme is relatively straight forward. They begin by creating a silicon membrane–a one dimensional silicon crystal about 200 nanometres thick. They engineer properties of this membrane such to create a kind of waveguide that steers phonons.
They create a phonon by zapping this membrane with a laser, which sends a quantum packet of vibration through the lattice.
The equivalent of an atom trapped in an optical cavity is single atom of boron or aluminium which distorts the lattice. It is this distortion that interacts with the photon.
However, physicists can use an external magnetic field to make the distortion take several different energy levels. This changes the way the phonon interacts in a way that processes the information it carries.
Finally, the phonon passes into another region of the silicon lattice with a band gap that has been engineered to convert the phonon into a photon, which can then be measured.
Circuit-QED has revolutionized the field of cavity-QED (cQED) providing a stable platform for light-matter interaction in the microwave regime along with large coupling strengths and solid state integrability. Progress in the field has enabled potential applications such as single microwave photon sources and quantum logic gates on a chip. In an ideal crystal environment, phonons may play a role analogous to photons, though they propagate with the much slower speed of sound. That acoustic phonons can be quantum coherent has been explored in a number of novel architectures, allowing seminal experiments in nanomechanical cooling trapping
of phonons in artificial phononic bandgap cavities photon translation via phonons, and indirect qubit-phonon coupling. What is missing to complete the analogy for
phonons is a non-linear element similar to an atom in cQED.Such an element has been proposed recently where a single donor in silicon (P:Si or Li:Si) couples directly to confined phonons to form a hybrid state: a phoniton (direct analog of the polariton in cQED). The impurity-phonon interaction may
be large due to large deformation potential matrix elements. The proposed system, however, requires very high frequencies (a few hundred GHz) and can be difficult
to integrate with other phonon components. While other impurities such as in diamond or in III-V semiconductors are possible, a practical system in silicon would be highly desirable given recent demonstrations of high-Q cavities in silicon
nanostructures, silicon’s investment in materials quality, and compatibility with CMOS technology and silicon photonics.
Key rates and parameters for circuit-QED (1D cavity), Quantum dot(QD)-QED22 (InAs QD embedded in a GaAs photonic crystal) vs. the B:Si phoniton system in a patterned Si membrane (of thickness d = 200nm) phononic bandgap cavity; we show calculations for maximal coupling at frequencies of 1GHz, 4GHz, 8GHz, and 14GHz, for cavity volume V = dl^2 and Q = 10^5. The limiting frequency for strong dispersive coupling is reached at 21GHz, when c = G. Number of Rabi flops, dispersive coupling, and dressed state’s resolution parameters, 2c=G, 2c=k, are comparable to that in circuit-QED and significantly surpass semiconductor quantum dots.
Conclusion
We have introduced a system that allows for on-chip manipulation of coherent acoustic phonons via coupling to acceptor qubit states in a nanomechanical
cavity. Hybridization of the phonon-acceptor system and strong dispersive coupling are both possible with comparable parameters to circuit-QED and far surpassing
semiconductor quantum dot QED. The phoniton component can be incorporated in more complex systems such as with phonon-photon interfaces to photonics and in arrays
of other phoniton systems for engineered many-body phonon devices. From the perspective of qubits, the isolated acceptor provides a potentially robust two-level system for quantum information processing. The phoniton component described here offers an avenue for phonon dispersive readout of acceptor qubit states and the potential for spin qubit-to-photon conversion in silicon – which would be a seminal accomplishment.
If you liked this article, please give it a quick review on ycombinator or StumbleUpon. Thanks
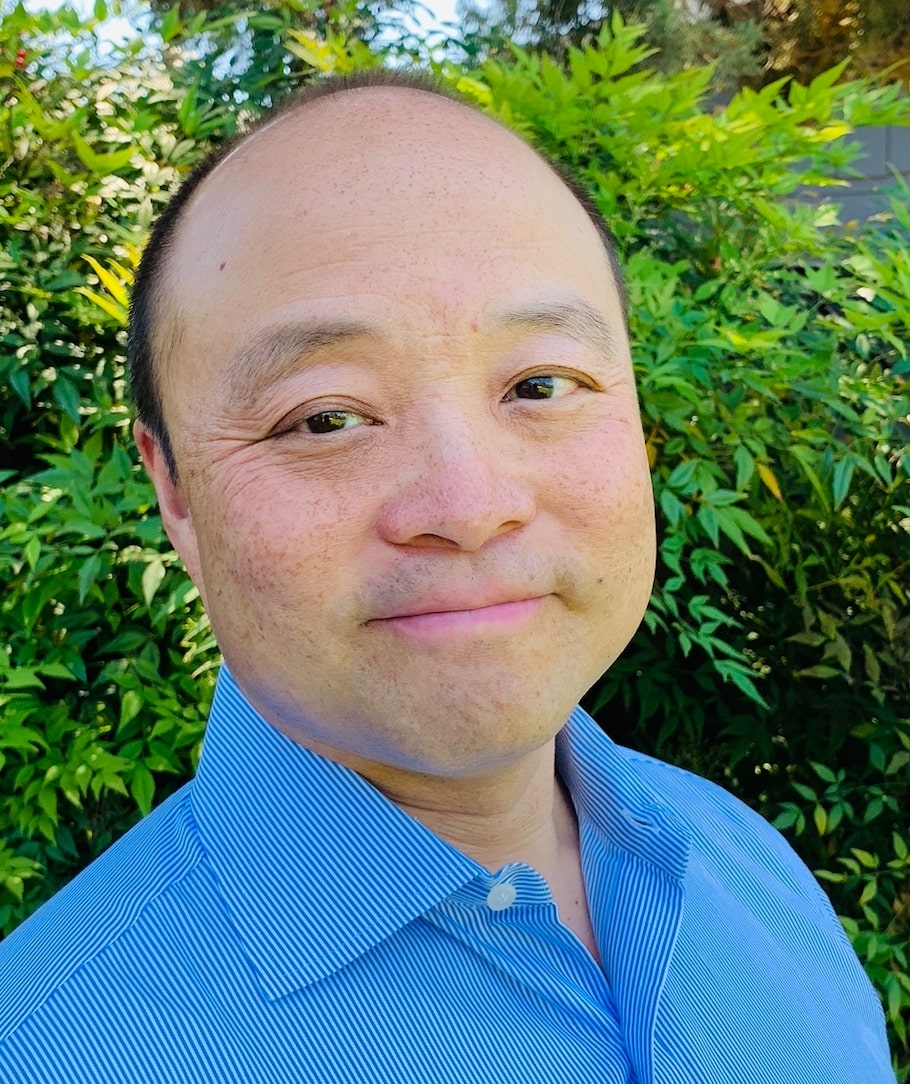
Brian Wang is a Futurist Thought Leader and a popular Science blogger with 1 million readers per month. His blog Nextbigfuture.com is ranked #1 Science News Blog. It covers many disruptive technology and trends including Space, Robotics, Artificial Intelligence, Medicine, Anti-aging Biotechnology, and Nanotechnology.
Known for identifying cutting edge technologies, he is currently a Co-Founder of a startup and fundraiser for high potential early-stage companies. He is the Head of Research for Allocations for deep technology investments and an Angel Investor at Space Angels.
A frequent speaker at corporations, he has been a TEDx speaker, a Singularity University speaker and guest at numerous interviews for radio and podcasts. He is open to public speaking and advising engagements.