The most observable leakage radiation from an advanced civilization may well be from the use of power beaming to transfer energy and accelerate spacecraft. Applications suggested for power beaming involve Earthto-space applications such as launching spacecraft to orbit, raising satellites to a higher orbit, and interplanetary concepts involving space-to-space transfers of cargo or passengers. We also quantify beam-driven launch to the outer solar system, interstellar precursors and ultimately starships. We estimate the principal observable parameters of power beaming leakage. Extraterrestrial civilizations would know their power beams could be observed, and so could put a message on the power beam and broadcast it for our receipt at little additional energy or cost. By observing leakage from power beams we may find a message embedded on the beam. Recent observations of the anomalous star KIC 8462852 by the Allen Telescope Array (ATA) set some limits on extraterrestrial power beaming in that system. We show that most power beaming applications commensurate with those suggested for our solar system would be detectable if using the frequency range monitored by the ATA, and so the lack of detection is a meaningful, if modest, constraint on extraterrestrial power beaming in that system. Until more extensive observations are made, the limited observation time and frequency coverage are not sufficiently broad in frequency and duration to produce firm conclusions.
Interplanetary logistics
A number of higher-velocity power beaming applications have been quantified for fast transit of the solar system – Mars, Jupiter, Kuiper Belt, Plutinos, Pluto and the Heliopause. An attractive interplanetary mission could be the rapid delivery of critical payloads within the solar system. For example, such emergencies as crucial equipment failures and disease outbreaks can make prompt delivery of small mass payloads to, e.g., Mars colonies, an imperative. Lasers or microwaves accelerate such urgent cargo with sail spacecraft at fast boost for a few hours of propulsion to speeds of 100 − 200 km/sec. The craft then coasts at constant high speed until decelerating for a few hours into Mars orbit (probably by a decelerating beam system like the one which launched it), giving a 10 day transit time (Meyer et al. 1985). This method has been extended to missions with 5 gee acceleration near Earth (Benford & Benford 2006). Using a ground station, acceleration occurs for a couple of hours for a 100 kg payload. Guillochon & Loeb (2015) have quantified a strategy for detecting leakage transients from such ETI interstellar logistics. They estimate that if we monitor continuously, the probability of detection would be on the order of 1% per planetary conjunction event. They state that “for a five-year survey with ∼ 10 conjunctions per system, about 10 multiply-transiting, inhabited systems would need to be tracked to guarantee a detection” with our existing radio telescopes.
Arxiv – Power Beaming Leakage Radiation as a SETI Observable
Interstellar Probes
Interstellar Probes are solar/interstellar boundary missions out to ∼ 1000 AU. The penultimate is the interstellar precursor mission. For this mission class, operating at high acceleration, the sail size can be reduced to less than 100 meters and accelerating power ∼ 100 MW focused on the sail. At 1 GW, sail size extends to 1 km and super-light probes reach velocities of 300 km/s (63 AU/year) for very fast missions of about 10 year duration (Benford & Benford 2006).
Starships: the biggest and grandest missions
Concepts of this sort require very large transmitter antenna/lens and sail (e.g., 1,000 km diameters for missions to 40 ly). A Space Solar Power (SSP; see below) station radiates a microwave beam to a perforated sail made of carbon nanotubes with lattice scale less than the microwave wavelength. The sizes of the first mission concepts were enormous, with sails on 1000 km scales (Forward 1984, 1985). Landis (1999) and Frisbee (2009) found ways to reduce it dramatically to 1-10 km. Systems were further optimized, with higher peak power of ∼ 10TW and smaller vehicle size of ∼ 0.1 − 1 km for the sail, requiring ∼ 100 km antenna array aperture (Dickinson 2001; Long 2011). Later concepts developed cost-optimized systems (Benford 2013).
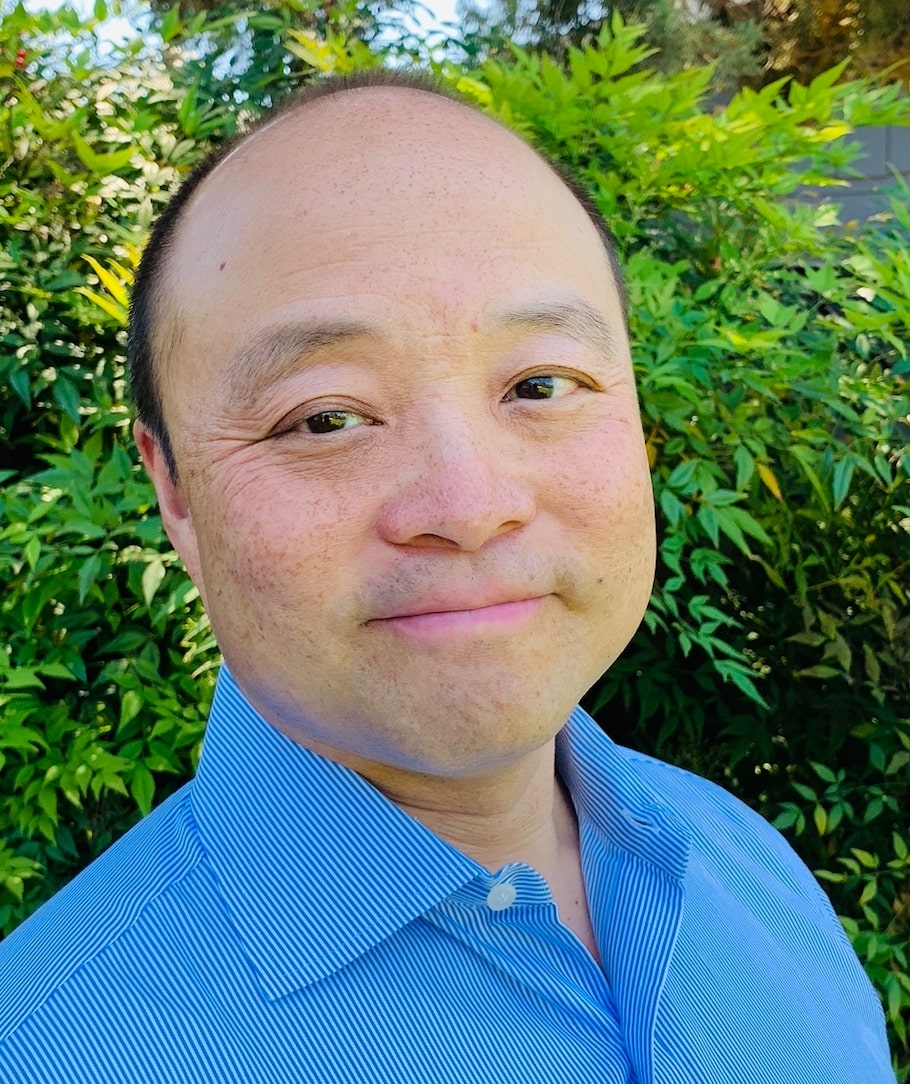
Brian Wang is a Futurist Thought Leader and a popular Science blogger with 1 million readers per month. His blog Nextbigfuture.com is ranked #1 Science News Blog. It covers many disruptive technology and trends including Space, Robotics, Artificial Intelligence, Medicine, Anti-aging Biotechnology, and Nanotechnology.
Known for identifying cutting edge technologies, he is currently a Co-Founder of a startup and fundraiser for high potential early-stage companies. He is the Head of Research for Allocations for deep technology investments and an Angel Investor at Space Angels.
A frequent speaker at corporations, he has been a TEDx speaker, a Singularity University speaker and guest at numerous interviews for radio and podcasts. He is open to public speaking and advising engagements.