Researchers at the University of Gothenburg and the University of Iceland are researching a new type of nuclear fusion process. This produces almost no neutrons but instead fast, heavy electrons (muons), since it is based on nuclear reactions in ultra-dense heavy hydrogen (deuterium).
The new fusion process can take place in relatively small laser-fired fusion reactors fuelled by heavy hydrogen (deuterium). It has already been shown to produce more energy than that needed to start it. Heavy hydrogen is found in large quantities in ordinary water and is easy to extract. The dangerous handling of radioactive heavy hydrogen (tritium) which would most likely be needed for operating large-scale fusion reactors with a magnetic enclosure in the future is therefore unnecessary.
” A considerable advantage of the fast heavy electrons produced by the new process is that these are charged and can therefore produce electrical energy instantly. The energy in the neutrons which accumulate in large quantities in other types of nuclear fusion is difficult to handle because the neutrons are not charged. These neutrons are high-energy and very damaging to living organisms, whereas the fast, heavy electrons are considerably less dangerous.”
Both heating generators and generators for electricity could be developed within a few years, according to research that has primarily been conducted at the University of Gothenburg.
Neutrons are difficult to slow down or stop and require reactor enclosures that are several metres thick. Muons – fast, heavy electrons – decay very quickly into ordinary electrons and similar particles.
Research shows that far smaller and simpler fusion reactors can be built. The next step is to create a generator that produces instant electrical energy.
Highlights
• Spontaneous high-energy particles from D(0) are detected by energy spectroscopy.
• These high-energy unstable particles give linear Kurie plots, indicating beta decay.
• The signal due to the unstable particles is strongly increased by glass converters.
• Spontaneous line-spectra are observed in the energy spectra.
• The signal may be due to spontaneous nuclear processes in D(0).
Abstract
High-energy particles are detected from spontaneous processes in an ultra-dense deuterium D(0) layer. Intense distributions of such penetrating particles are observed using energy spectroscopy and glass converters. Laser-induced emission of neutral particles with time-of-flight energies of 1–30 MeV u−1 was previously reported in the same system. Both spontaneous line-spectra and a spontaneous broad energy distribution similar to a beta-decay distribution are observed. The broad distribution is concluded to be due to nuclear particles, giving straight-line Kurie-like plots. It is observed even at a distance of 3 meters in air and has a total rate of 10^7–10^10 per second. If spontaneous nuclear fusion or other nuclear processes take place in D(0), it may give rise to the high-energy particle signal. Low energy nuclear reactions (LENR) and so called cold fusion may also give rise to such particles.
AIP Advances – Heat generation above break-even from laser-induced fusion in ultra-dense deuterium
Previous results from laser-induced processes in ultra-dense deuterium D(0) give conclusive evidence for ejection of neutral massive particles with energy over 10 MeV u−1. Such particles can only be formed from nuclear processes like nuclear fusion at the low laser intensity used. Heat generation is of interest for future fusion energy applications and has now been measured by a small copper (Cu) cylinder surrounding the laser target. The temperature rise of the Cu cylinder is measured with an NTC resistor during around 5000 laser shots per measured point. No heating in the apparatus or the gas feed is normally used. The fusion process is suboptimal relative to previously published studies by a factor of around 10. The small neutral particles H N (0) of ultra-dense hydrogen (size of a few pm) escape with a substantial fraction of the energy. Heat loss to the D2 gas (at less than 1 mbar pressure) is measured and compensated for under various conditions. Heat release of a few W is observed, at up to 50% higher energy than the total laser input thus a gain of 1.5. This is uniquely high for the use of deuterium as fusion fuel. With a slightly different setup, a thermal gain of 2 is reached, thus clearly above break-even for all neutronicity values possible. Also including the large kinetic energy which is directly measured for MeV particles leaving through a small opening gives a gain of 2.3. Taking into account the lower efficiency now due to the suboptimal fusion process, previous studies indicate a gain of at least 20 during long periods.
The laser-induced nuclear fusion process in ultra-dense deuterium D(0) gives a heating power at least a factor of 2 larger than the laser power into the apparatus, thus clearly above break-even. This is found with 100-200 mJ laser pulse-energy into the apparatus. No heating is used in the system, to minimize problems with heat transfer and gas transport. This gives sub-optimal conditions, and the number of MeV particles (and thus their energy) created in the fusion process is a factor of 10 below previous more optimized conditions. Several factors lead to lower measured heat than the true value, and the results found are thus lower limits to the real performance. With the optimum source conditions used previously, a gain of 20 is likely also for longer periods.
Muons are conventionally measured by a plastic scintillator–photomultiplier detector. Muons from processes in ultra-dense hydrogen H(0) are detected here by a novel type of converter in front of a photomultiplier. The muon detection yield can be increased relative to that observed with a plastic scintillator by at least a factor of 100, using a converter of metal, semiconductor (Ge), or glass for interaction with the muons penetrating through the metal housing of the detector. This detection process is due to transient formation of excited nuclei by the well-known process of muon capture, giving beta decay. The main experimental results shown here are in the form of beta electron energy spectra detected directly by the photomultiplier. Events which give a high-energy tail in the energy spectra are probably due to gamma photons from the muons. Sharp and intense x-ray peaks from a muonic aluminium converter or housing material are observed. The detection conversion in glass and Ge converters has a time constant of the order of many minutes to reach the final conversion level, while the process in metal converters is stabilized faster. The time constants are not due to lifetimes of the excited nuclei or neutrons but are due to internal charging in the insulating converter material. Interaction of this charging with the high voltage in the photomultiplier is observed.
Principle of the copper (Cu) cylinder setup. The temperature is read from the resistance of an NTC resistor fastened to the outer surface of the Cu cylinder as shown in the left-hand figure. The chamber is pumped continuously at the deuterium pressure of ≤ 1.0 mbar. No heat (left figure) is used in most experiments, while the highest gain is found for the construction to the right where D(0) from a slightly heated source is collected on the Cu surface.
Ultradense Deuterium Production announcement from 2009
Ultradense deuterium is a hundred thousand times heavier than water and more dense than the core of the Sun. The University of Gothenburg had announced microscopic production back in 2009.
UDD is an exotic form of metallic hydrogen (Rydberg matter) and is supposedly found along with the regular metallic hydrogen in the experiments performed by Dr. Holmlid and his group at the University of Gothenburg. The list of its exotic properties is long and it includes superconductivity and superfluidity at room temperature as well as its primary characteristic: enormous density. The estimates of its density vary from 1028 to 1029 cm-3 based on theoretical predictions and experimental observations. This density level is roughly 150 times higher than the originally predicted National Ignition Facility ICF target at peak compression. The enormous density would imply that, as shown by Winterberg, a very low energy input is required for the ignition of the fusion target, while completely circumventing the need for target compression and thus a series of physics issues that come along with it. The numbers estimate that a single PW-scale, 3 kJ laser beam would be sufficient to start the fusion reaction
SOURCES – University of Gothenburg, AIP Advances, Review of Scientific Instruments, International Journal of Hydrogen Energy
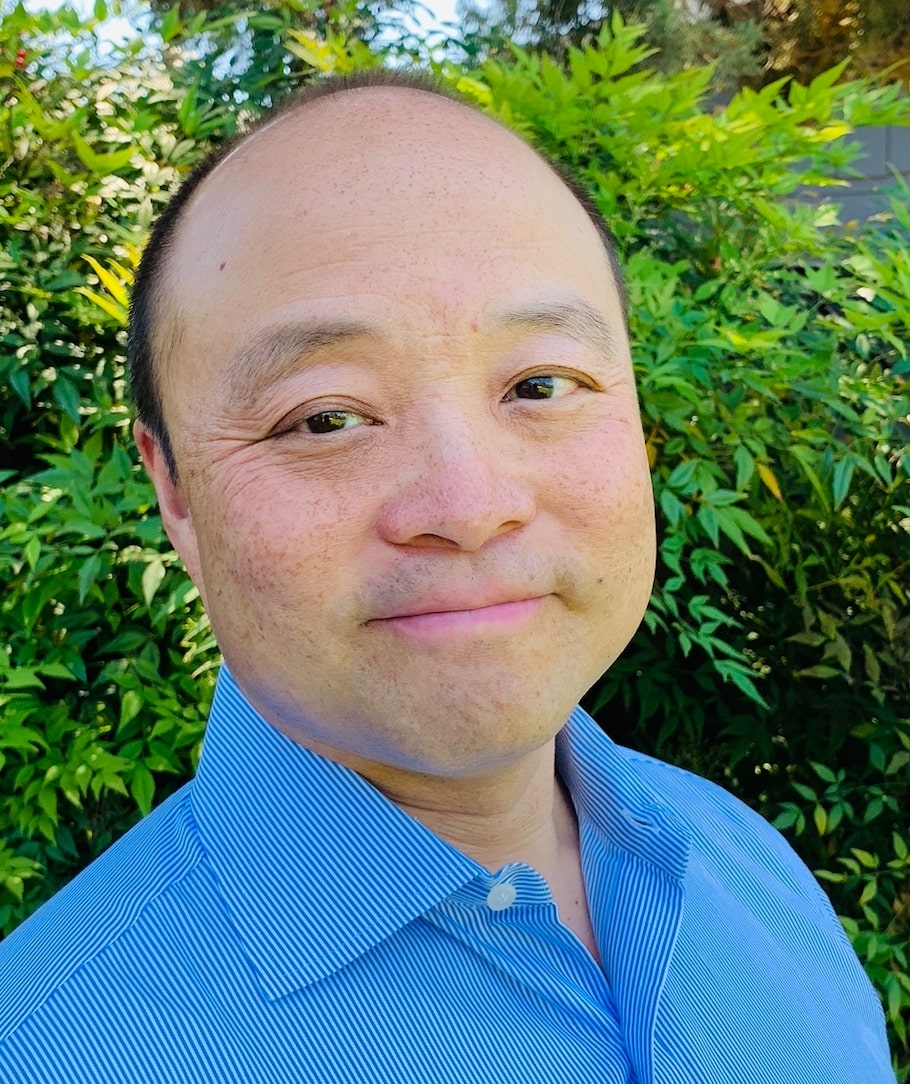
Brian Wang is a Futurist Thought Leader and a popular Science blogger with 1 million readers per month. His blog Nextbigfuture.com is ranked #1 Science News Blog. It covers many disruptive technology and trends including Space, Robotics, Artificial Intelligence, Medicine, Anti-aging Biotechnology, and Nanotechnology.
Known for identifying cutting edge technologies, he is currently a Co-Founder of a startup and fundraiser for high potential early-stage companies. He is the Head of Research for Allocations for deep technology investments and an Angel Investor at Space Angels.
A frequent speaker at corporations, he has been a TEDx speaker, a Singularity University speaker and guest at numerous interviews for radio and podcasts. He is open to public speaking and advising engagements.