Exoplanets are detected—by measuring the wobble their gravity causes in their host stars, or by noting the slight decline in a star’s brightness as a planet passes in front of it—yield little detail. Using them, astronomers can infer such basics as a planet’s size, mass and orbit. Occasionally, they can interrogate starlight that has traversed a planet’s atmosphere about the chemistry of its air.
What would help is the ability to take pictures of planets directly. Such images could let astronomers deduce a world’s surface temperature, analyse what that surface is made from and even—if the world were close enough and the telescope powerful enough—get a rough idea of its geography. Gathering the light needed to create such images is hard. The first picture of an extrasolar world, 2M1207b, 170 light-years away, was snapped in 2004, but the intervening dozen years have seen only a score or so of others join it in the album. That should soon change, though, as new instruments both on the ground and in space add to the tally. And a few of the targets of these telescopes may be the sorts of planets that have the best chance of supporting life, namely Earth-sized worlds at the right distance from sun-like stars, in what are known as those stars’ habitable zones—places where heat from the star might be expected to stop water freezing without actually boiling it.
Taking pictures of exoplanets is hard for two reasons. One is their distance. The other is that they are massively outshone by their host stars.
Interstellar distances do not just make objects faint. They also reduce the apparent gap between a planet and its host, so that it is hard to separate the two in a photograph. Such apparent gaps are measured in units called arc-seconds (an arc-second is a 3,600th of a degree). This is about the size of an American dime seen from four kilometers away. The exoplanet closest to Earth orbits Proxima Centauri, the sun’s stellar neighbor. Yet despite its proximity (4.25 light-years) the angular gap between this planet and its star is a mere 0.038 arc-seconds, according to Beth Biller, an exoplanet specialist at the University of Edinburgh. Separating objects which appear this close together requires a pretty big telescope.
The second problem, glare, is best dealt with by inserting an opaque disc called a coronagraph into a telescope’s optics. A coronagraph’s purpose is to block light coming directly from a star while permitting any that is reflected from planets orbiting that star to shine through. This palaver is necessary because, as a common analogy puts it, photographing an exoplanet is like trying to take a picture, from thousands of kilometers away, of a firefly buzzing around a lighthouse. Seen from outside the solar system, Earth would appear to be a ten-billionth as bright as the sun.
Exoplanets that have had their photographs taken so far are ones for which these problems are least troublesome—gigantic orbs (which thus reflect a lot of light) circling at great distances (maximising angular separation) from dim hosts (minimising glare).
The three most capable exaoplanet telescopes are
1. the Gemini Planet Imager, attached to the southern Gemini telescope, in Chile;
2. the Spectro-Polarimetric High-Contrast Exoplanet Research Instrument on the Very Large Telescope, a European machine also in Chile; and
3. the Subaru Coronagraphic Extreme Adaptive Optics Device on the Subaru telescope, a Japanese machine on Hawaii.
All of those telescopes sport a mirror more than eight meters across, making them some of the biggest in the world, and their planet-photographing attachments are fitted with the most sophisticated coronagraphs available. The result is that the Subaru device, for example, can take pictures of giant planets that orbit their stars slightly closer in than Jupiter orbits the sun.
They are still limited to photographing gas giants. To take snaps of the next-smallest class of planets (so-called “ice giants” like Neptune and Uranus), and the class after that (large, rocky planets called “super-Earths” that have no analogue in the solar system), will require even more potent instruments.
What big ground based telescopes are being built or are funded which will provide a better look a this and other earthlike exoplanets ?
* James Webb Space Telescope (2018)
* 24 meter ground based telescope, Giant Megallan (2022-2025)
* 39 meter ground based Extremely Large Telescope (2024-2027)
* 30 Meter Telescope (permit delays, around 2022-2025 if resolved)
The James Webb space telescope should be deployed in 2018
JWST will have the capability to detect key markers that could indicate the presence of a climate like our own when looking at Earth-sized planets around stars that are smaller and redder than our sun (like Proxima b). A study shows that the James Webb Space Telescope – Hubble’s successor could distinguish between an Earth and a Venus orbiting a cool, red star not too far away. But making that observation wouldn’t be easy.
The 24 meter Giant Magellan Telescope should also be operating around 2022-2025. The GMT will have absolute magnitude capability of 29.
Targets for direct imaging exoplanets fall into a few distinct classes:
• Planets still embedded in their parent disks (age = 1 – 10Myr, at 30 – 150pc).
• Young (0.1 – 1Gyr), nearby (3 – 50pc) gas-giant planets, which are intrinsically bright in the near-infrared due to their on-going gravitational contraction and,
• Older (over 1Gyr) planets detectable via their thermal infrared emission or reflected light.
The GMT will provide high contrast, high resolution imaging capabilities in the near and mid-infrared enabling the detection of exoplanets in each of these categories.
One of the technical goals of the GMT is to detect objects more than one million times fainter than the host star at angular separations corresponding to 1.5 λ/D to 20 λ/D
Extremely Large Telescope
A 40-meter-class mirror will allow the study of the atmospheres of extrasolar planets.
The telescope’s segmented mirror will be 39.3 meters in diameter and will gather 15 times more light than the largest optical telescopes operating at the time of its development. The telescope has an innovative five-mirror design that includes advanced adaptive optics to correct for the turbulent atmosphere, giving exceptional image quality
Near Term Space Telescopes
The James Webb space telescope is scheduled for launch in 2018 and which boasts both a mirror 6.5 meters across and a reasonably capable coronagraph, should be able to snap pictures of some large, nearby worlds. It will be able to sniff the atmospheres of many more, analysing starlight that has passed through those atmospheres on its way to Earth.
The Wide Field Infrared Survey Telescope (WFIRST) is a future infrared space observatory. On February 17, 2016, WFIRST was formally designated as a mission by NASA.
In fiscal year 2014, Congress provided $56 million for WFIRST, and in 2015 Congress provided $50 million.The fiscal year 2016 spending bill provided $90 million for WFIRST. The WFIRST mission entered the “formulation phase” in February 2016.
WFIRST is on a plan for a mid-2020s launch. The total cost of WFIRST is expected at more than $2 billion; NASA’s latest estimate is around $2.0 billion in 2010 dollars, which corresponds to around $2.7 billion in real year (inflation-adjusted) dollars
Crowdfunded innovation
Project Blue will use a mirror between 30 and 45 centimeters across and cost only $50 million. It will have a computer-run “multi-star wavefront controlled” mirror. This will draw on a technology already fitted to ground-based telescopes, called adaptive optics, in which portions of the mirror are subtly deformed in order to sculpt incoming light.
In combination with a coronagraph the wavefront controller will let the telescope blot out the light not only of Alpha Centauri A, but also of Alpha Centauri B, a companion even closer to it than Proxima Centauri is. The plan is to take thousands of pictures over the course of several years. By combining these and looking for persistent signals—particularly ones that appear to follow plausible orbits—computers should be able to pluck any planets from the noise.
Examining the spectrum of light from them would reveal what their atmospheres and surfaces were made from, including any chemicals—such as oxygen and methane—that might suggest the presence of life. It might even be possible to detect vegetation, or its alien equivalent, directly. The length of a planet’s day could be inferred by watching for regular changes in light as its revolution about its axis caused continents and seas to become alternately visible and invisible. Longer-term variations might reveal planetary seasons; shorter-term, more chaotic ones might be evidence of weather.
If Project Blue works then this approach could enable exoplanet telescopes that are 20 to 100 times less expensive than traditional space telescopes.
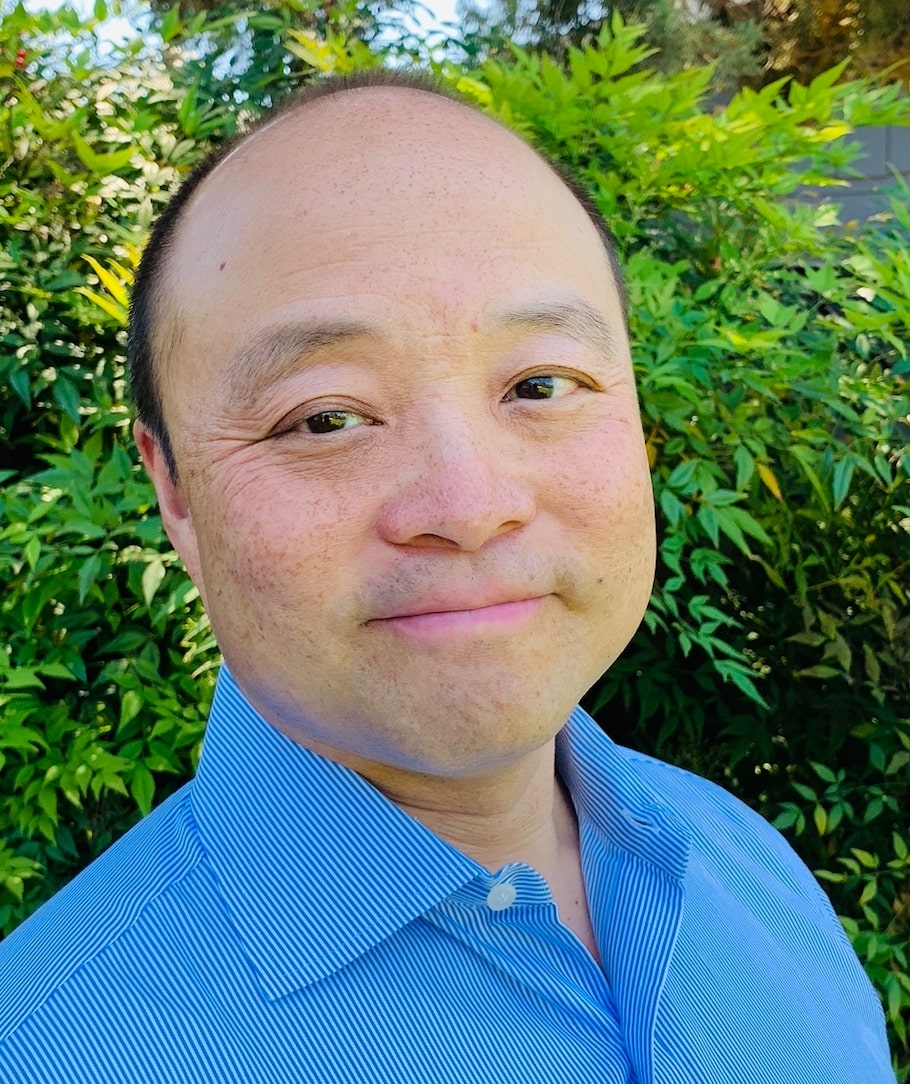
Brian Wang is a Futurist Thought Leader and a popular Science blogger with 1 million readers per month. His blog Nextbigfuture.com is ranked #1 Science News Blog. It covers many disruptive technology and trends including Space, Robotics, Artificial Intelligence, Medicine, Anti-aging Biotechnology, and Nanotechnology.
Known for identifying cutting edge technologies, he is currently a Co-Founder of a startup and fundraiser for high potential early-stage companies. He is the Head of Research for Allocations for deep technology investments and an Angel Investor at Space Angels.
A frequent speaker at corporations, he has been a TEDx speaker, a Singularity University speaker and guest at numerous interviews for radio and podcasts. He is open to public speaking and advising engagements.