Stanford Linear Accelerator Lab (SLAC) scientists were able to observe “diamond rain” for the first time as it formed in high-pressure conditions. Extremely high pressure squeezes hydrogen and carbon found in the interior of these planets to form solid diamonds that sink slowly down further into the interior.
The glittering precipitation has long been hypothesized to arise more than 5,000 miles below the surface of Uranus and Neptune, created from commonly found mixtures of just hydrogen and carbon. The interiors of these planets are similar—both contain solid cores surrounded by a dense slush of different ices. With the icy planets in our solar system, “ice” refers to hydrogen molecules connected to lighter elements, such as carbon, oxygen and/or nitrogen.
They were able to see that nearly every carbon atom of the original plastic was incorporated into small diamond structures up to a few nanometers wide. On Uranus and Neptune, the study authors predict that diamonds would become much larger, maybe millions of carats in weight. Researchers also think it’s possible that over thousands of years, the diamonds slowly sink through the planets’ ice layers and assemble into a thick layer around the core.
“Previously, researchers could only assume that the diamonds had formed,” said Dominik Kraus, scientist at Helmholtz Zentrum Dresden-Rossendorf and lead author on the publication. “When I saw the results of this latest experiment, it was one of the best moments of my scientific career.”
A cutaway depicts the interior of Neptune (left). In an experiment conducted at the Linac Coherent Light Source, the team studied a plastic simulating compounds formed from methane—a molecule with just one carbon bound to four hydrogen atoms that causes the distinct blue cast of Neptune. Methane forms hydrocarbon (hydrogen and carbon) chains that respond to high pressure and temperature to form “diamond rain” in the interiors of icy giant planets like Neptune. The scientists were able to recreate similar conditions using high-powered optical lasers and watch the small diamonds form in real time with X-rays. (Greg Stewart/SLAC National Accelerator Laboratory)
Earlier experiments that attempted to recreate diamond rain in similar conditions were not able to capture measurements in real time, because we currently can create these extreme conditions under which tiny diamonds form only for very brief time in the laboratory. The high-energy optical lasers at MEC combined with LCLS’s X-ray pulses—which last just femtoseconds, or quadrillionths of a second—allowed the scientists to directly measure the chemical reaction.
Other prior experiments also saw hints of carbon forming graphite or diamond at lower pressures than the ones created in this experiment, but with other materials introduced and altering the reactions.
The results presented in this experiment is the first unambiguous observation of high-pressure diamond formation from mixtures and agrees with theoretical predictions about the conditions under which such precipitation can form and will provide scientists with better information to describe and classify other worlds.
Turning Plastic Into Diamond
In the experiment, plastic simulates compounds formed from methane—a molecule with just one carbon bound to four hydrogen atoms that causes the distinct blue cast of Neptune.
The team studied a plastic material, polystyrene, that is made from a mixture of hydrogen and carbon, key components of these planets’ overall chemical makeup.
In the intermediate layers of icy giant planets, methane forms hydrocarbon (hydrogen and carbon) chains that were long hypothesized to respond to high pressure and temperature in deeper layers and form diamond rain.
The researchers used high-powered optical laser to create pairs of shock waves in the plastic with the correct combination of temperature and pressure. The first shock is smaller and slower and overtaken by the stronger second shock. When the shock waves overlap, that’s the moment the pressure peaks and when most of the diamonds form, Kraus said.
During those moments, the team probed the reaction with pulses of X-rays from LCLS that last just 50 femtoseconds. This allowed them to see the small diamonds that form in fractions of a second with a technique called femtosecond X-ray diffraction. The X-ray snapshots provide information about the size of the diamonds and the details of the chemical reaction as it occurs.
“For this experiment, we had LCLS, the brightest X-ray source in the world,” said Siegfried Glenzer, professor of photon science at SLAC and a co-author of the paper. “You need these intense, fast pulses of X-rays to unambiguously see the structure of these diamonds, because they are only formed in the laboratory for such a very short time.”
The Matter in Extreme Conditions instrument at SLAC gives scientists the tools to investigate the extremely hot, dense matter at the centers of stars and giant planets. These experiments could help researchers design new materials with enhanced properties and recreate the nuclear fusion process that powers the sun. (SLAC National Accelerator Laboratory)
Information from studies like this one about how elements mix and clump together under pressure in the interior of a given planet can change the way scientists calculate the relationship between mass and radius, allowing scientists to better model and classify individual planets. The falling diamond rain also could be an additional source of energy, generating heat while sinking towards the core.
“We can’t go inside the planets and look at them, so these laboratory experiments complement satellite and telescope observations,” Kraus said.
The researchers also plan to apply the same methods to look at other processes that occur in the interiors of planets.
In addition to the insights they give into planetary science, nanodiamonds made on Earth could potentially be harvested for commercial purposes—uses that span medicine, scientific equipment and electronics. Currently, nanodiamonds are commercially produced from explosives; laser production may offer a cleaner and more easily controlled method.
Abstract
The effects of hydrocarbon reactions and diamond precipitation on the internal structure and evolution of icy giant planets such as Neptune and Uranus have been discussed for more than three decades1. Inside these celestial bodies, simple hydrocarbons such as methane, which are highly abundant in the atmospheres, are believed to undergo structural transitions that release hydrogen from deeper layers and may lead to compact stratified cores. Indeed, from the surface towards the core, the isentropes of Uranus and Neptune intersect a temperature–pressure regime in which methane first transforms into a mixture of hydrocarbon polymers, whereas, in deeper layers, a phase separation into diamond and hydrogen may be possible. Here we show experimental evidence for this phase separation process obtained by in situ X-ray diffraction from polystyrene (C8H8) n samples dynamically compressed to conditions around 150 GPa and 5,000 K; these conditions resemble the environment around 10,000 km below the surfaces of Neptune and Uranus. Our findings demonstrate the necessity of high pressures for initiating carbon–hydrogen separation3 and imply that diamond precipitation may require pressures about ten times as high as previously indicated by static compression experiments4,8,10. Our results will inform mass–radius relationships of carbon-bearing exoplanets11, provide constraints for their internal layer structure and improve evolutionary models of Uranus and Neptune, in which carbon–hydrogen separation could influence the convective heat transport.
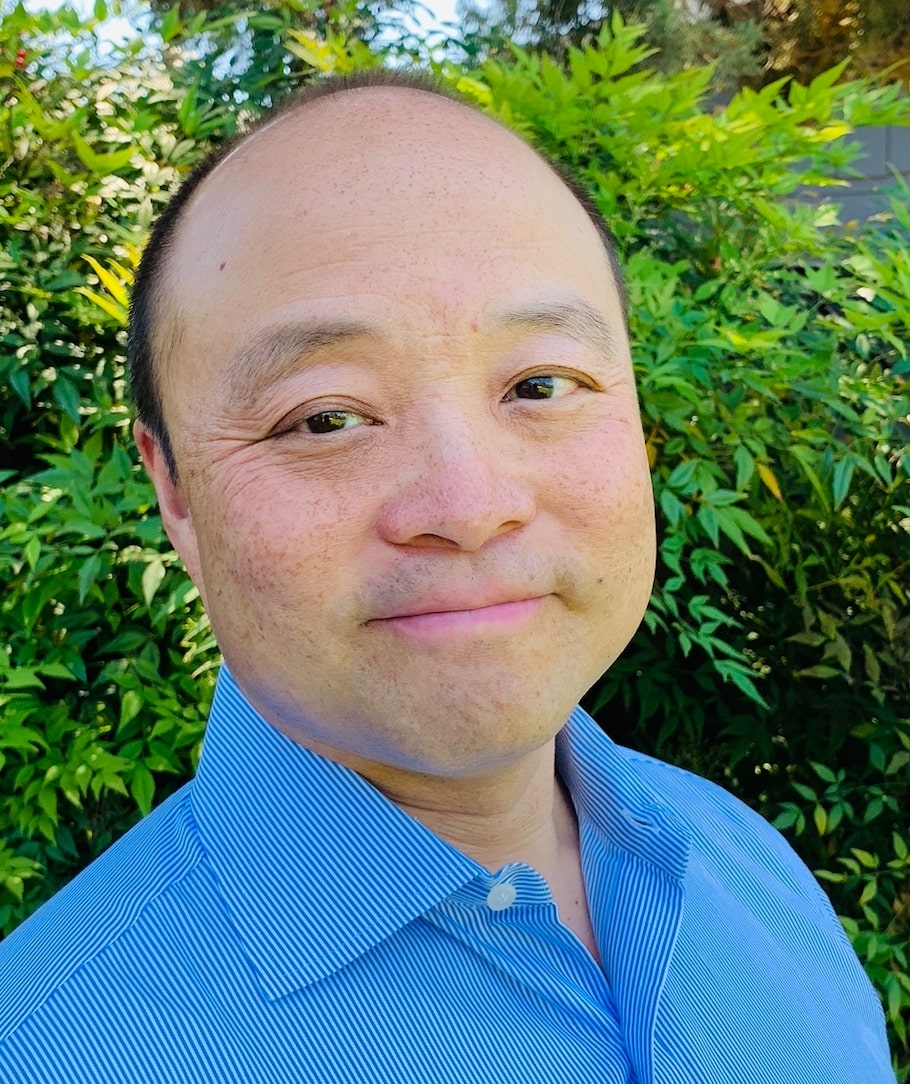
Brian Wang is a Futurist Thought Leader and a popular Science blogger with 1 million readers per month. His blog Nextbigfuture.com is ranked #1 Science News Blog. It covers many disruptive technology and trends including Space, Robotics, Artificial Intelligence, Medicine, Anti-aging Biotechnology, and Nanotechnology.
Known for identifying cutting edge technologies, he is currently a Co-Founder of a startup and fundraiser for high potential early-stage companies. He is the Head of Research for Allocations for deep technology investments and an Angel Investor at Space Angels.
A frequent speaker at corporations, he has been a TEDx speaker, a Singularity University speaker and guest at numerous interviews for radio and podcasts. He is open to public speaking and advising engagements.