A team at the DIII-D National Fusion Facility led by a William & Mary physicist has made a significant advancement in physics understanding that represents a key step toward practical fusion energy.
The work, published in an article in the journal Nuclear Fusion, helps better explain the relationship among three variables – plasma turbulence, the transport of electrons through the plasma and electron density in the core. Because these factors are key elements of the fusion reaction, this understanding could significantly improve the ability to predict performance and efficiency of fusion plasmas, a necessary step toward achieving commercial fusion power plants.
They confirmed low collisionality improves electron density peaking through the formation of an internal barrier to particle movement through the plasma, which in turn altered the plasma turbulence. Previous work had suggested the effect might be due to plasma heating by neutral beam injection, but the experiments show that it was linked to particle transport and turbulence.
“We’ve known for some time that there is a relationship between core electron density, electron-ion collisions and particle movement in the plasma,” said William & Mary’s Saskia Mordijck, who led the multi-institutional research team at DIII-D. “Unfortunately, until now research has not been able to untangle that relationship from the other components that affect electron density patterns.”
DIII-D, which General Atomics operates as a national user facility for the Department of Energy’s Office of Science, is the largest magnetic fusion research facility in the country. It hosts researchers from more than 100 institutions across the globe, including 40 universities. The heart of the facility is a tokamak that uses powerful electromagnets to produce a doughnut-shaped magnetic vessel containment for confining a fusion plasma. In DIII-D, plasma temperatures more than 10 times hotter than the Sun are routinely achieved. At such extremely high temperatures, hydrogen isotopes can fuse together and release energy.
In a tokamak, fusion power is determined by temperature, plasma density and confinement time. Fusion gain, expressed as the symbol Q, is the ratio of fusion power to the input power required to maintain the reaction and is thus a key indicator of the device’s efficiency. At Q = 1, the breakeven point has been reached, but because of heat losses, self-sustaining plasmas are not reached until about Q = 5. Current systems have achieved extrapolated values of Q = 1.2. The ITER experiment under construction in France is expected to achieve Q = 10, but commercial fusion power plants will likely need to achieve even higher Q values to be economical.
Abstract
The results of the experimental dimensionless scan in this paper confirm that there is an increase in density peaking towards lower collisionality and that this can be partly linked to a shift in the turbulence regime from ITG towards TEM. However at the lowest collisionality, the changes in turbulence and transport are much more pronounced than expected from direct collisionality effect on the turbulence. In this paper, the collisionality is varied by a factor 5, while keeping other variables fixed. Additionally, a 3 Hz gas puff modulation is applied to modulate the electron density profile and extract the perturbed transport coefficients using two diagnostics. The transport analysis shows that the increase in density peaking at low ν * is linked to an increase in the inward particle pinch and not an increase in core fueling. These observations are not only in agreement with prior modeling scans of how turbulence changes as a function of collisionality and its impact upon the particle fluxes, but also with the multi-machine database (Fable E. et al 2010 Plasma Phys. Control. Fusion 52 015007) (Angioni C. et al 2003 Phys. Rev. Lett. 90 205003). The changes in turbulence across the collisionality scan were captured at large scale by the BES and at smaller scale by the DBS. A comparison with gradient-driven GENE simulations showed similar trends at both scales. Moreover, the changes observed in overall transport are in agreement with gradient-driven TGLF particle flux simulations. This indicates that TGLF/GENE when given the gradients as input, are able to reproduce the experimentally observed turbulence changes.
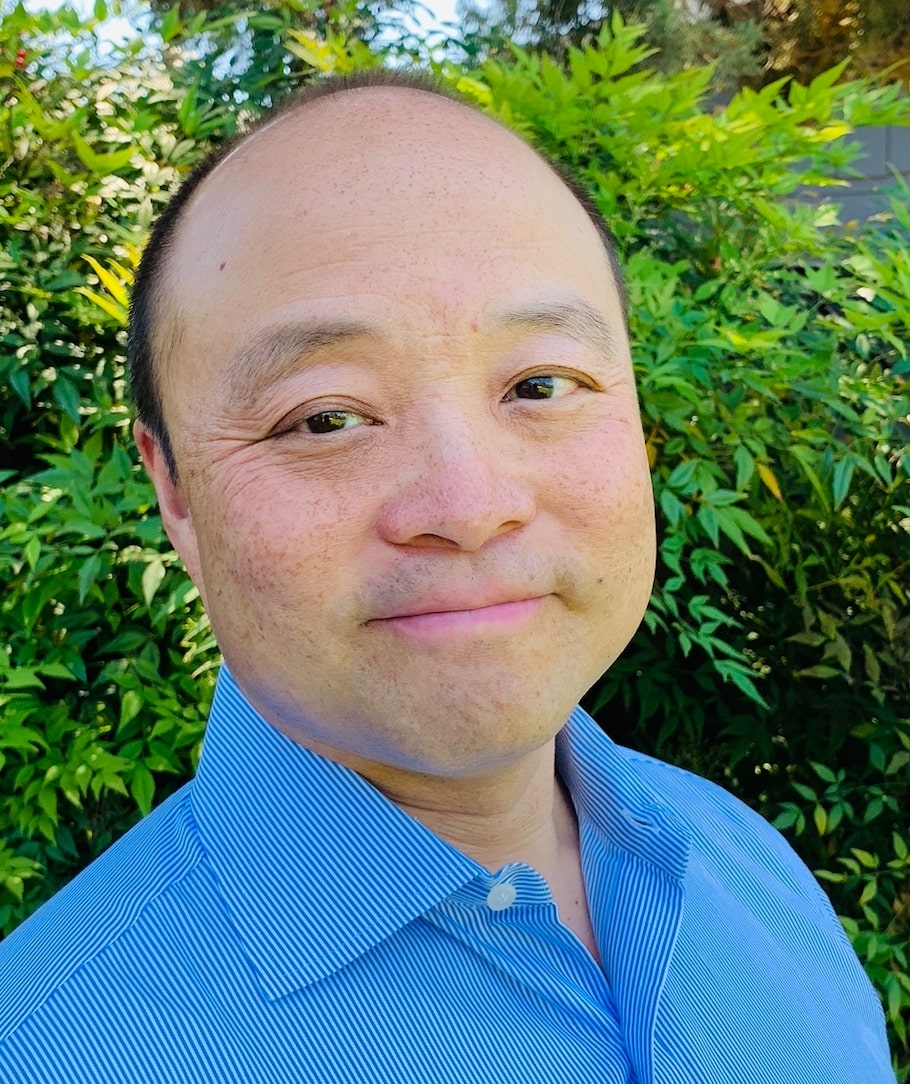
Brian Wang is a Futurist Thought Leader and a popular Science blogger with 1 million readers per month. His blog Nextbigfuture.com is ranked #1 Science News Blog. It covers many disruptive technology and trends including Space, Robotics, Artificial Intelligence, Medicine, Anti-aging Biotechnology, and Nanotechnology.
Known for identifying cutting edge technologies, he is currently a Co-Founder of a startup and fundraiser for high potential early-stage companies. He is the Head of Research for Allocations for deep technology investments and an Angel Investor at Space Angels.
A frequent speaker at corporations, he has been a TEDx speaker, a Singularity University speaker and guest at numerous interviews for radio and podcasts. He is open to public speaking and advising engagements.
And therefore …. ??
I suspect that a critical part of Musk’s success is choosing which fields to go into.
He wants a field where the current industrial standard is way, way behind the available tech, and so it just needs someone to start from a clean sheet and do it properly.
Here lies a 2019 publication from L.H. which provides a timeline and reference collection from the prior ten years.
https://iopscience.iop.org/article/10.1088/1402-4896/ab1276/pdf
The little bit I found on his work seems to relate hyperdense hydrogen to Rydberg matter. But Rydberg atoms have the electrons in very large orbitals & Rydberg matter is normally *low* density. Can you point me to something that explains this, to someone whose quantum mechanics is a bit rusty?
All this new tech and more could come from a DARPA-like ‘endless budget’ entity – “… The Endless Frontiers Act (S. 3832) proposes a major reorganization of NSF, creating a technology directorate that, within 4 years, would grow to more than four times the size of the entire agency’s existing $8 billion budget. NSF would be renamed the National Science and Technology Foundation…”
this is the third option.. not the evil-but-effective Market or the good-but-incompetent Bureaucrats, this is DARPA done broad spectrum – the value of re-establishing US world leadership in AI, supercomputing, quantum, replacing critical metals, fusion energy, etc., where all have fundamental physics/ mathematics under-pinnings not yet figured out – this is more than a top University lab could accomplish, greater than a skunkworks corporate department (if any still exist) could do. They key is whether they are DARPA secret or need to publish consistently, etc. Exciting.
It stands to reason that a breakthrough in fusion would attract space flight developers – the crux of achieving space flight was managed more than half a century ago though, it’s not surprising that they have been largely resting on their laurels to the point that it took a profit seeking entrepeneur to shake things up.
If a monied personality like Musk put their shoulders to the wheel on fusion I imagine some of those startups would get results much quicker, as it is they are just dipping their toes in with bit size milestones because getting the funding is such a pain.
The difference is that
— Fusion has a lot more startup competitors who think they can do it faster and cheaper than the big boys.
— Space flight, though with much fewer players, has actually crossed the critical threshold of working so that it is the basis of a well established profitable industry rather than a still hopeful research project.
Given that they both started as the outgrowth of advanced World War 2 weapons programs that achieved their initial success in the 1950s cold war it’s a pity the two programs stopped developing in parallel.
Have a glance at Sindre’s (norrønt ) work toward an advanced neutron source. Also, discussion of possible anti-neutron connection to laser irradiation of H(0).
Given the state of fusion, I’m rather crossing my fingers for Leif Holmlid’s results related to hyper-dense protium and deuterium to be correct and replicable by others (so far, it seems they are), so we may soon have a reliable and plentiful source of muons for much easier nuclear fusion.
And matter-antimatter annihilation-like amounts of energy from the same reaction, of course, that would be handy too.
The ITER shares much in common with NASA, such as a decades long timeline for results, an extravagant budget ten times the investment of competing systems, and a bloated employee roster.