NASA researchers have detected nuclear fusion in metal loaded with deuterium. A metal such as erbium is loaded with deuterium atoms (aka deuterons) packing the fuel a billion times denser than in magnetic confinement (tokamak) fusion reactors. In the new method, a neutron source accelerates (heats) deuterons until they start colliding with a neighboring deuteron. This causes D-D fusion reactions. The neutrons were created through photodissociation of deuterons via exposure to 2.9+MeV gamma (energetic X-ray) beam. Upon irradiation, some of the fuel deuterons dissociate resulting in both the needed energetic neutrons and protons. They measured nuclear fusion reactions and they saw the production of even more energetic neutrons which is evidence of boosted fusion reactions or screened Oppenheimer-Phillips (O-P) nuclear stripping reactions with the metal lattice atoms.
Either reaction could be scalable to useful levels of power. The goal will be to create power systems for long-duration space exploration missions or in-space propulsion. It could be developed into electrical power or creating medical isotopes for nuclear medicine.
The metal lattice, loaded with deuterium fuel, is at room temperature overall but the new method creates an energetic environment inside the lattice where individual atoms reach equivalent fusion-level kinetic energies.
Physical Review C – Nuclear fusion reactions in deuterated metals
Vladimir Pines, Marianna Pines, Arnon Chait, Bruce M. Steinetz, Lawrence P. Forsley, Robert C. Hendricks, Gustave C. Fralick, Theresa L. Benyo, Bayarbadrakh Baramsai, Philip B. Ugorowski, Michael D. Becks, Richard E. Martin, Nicholas Penney, and Carl E. Sandifer, II
Phys. Rev. C 101, 044609 – Published 20 April 2020
ABSTRACT
Nuclear fusion reactions of D-D are examined in an environment comprised of high density cold fuel embedded in metal lattices in which a small fuel portion is activated by hot neutrons. Such an environment provides for enhanced screening of the Coulomb barrier due to conduction and shell electrons of the metal lattice, or by plasma induced by ionizing radiation (γ quanta). We show that neutrons are far more efficient than energetic charged particles, such as light particles or heavy particles in transferring kinetic energy to fuel nuclei (D) to initiate fusion processes. It is well known that screening increases the probability of tunneling through the Coulomb barrier. Electron screening also significantly increases the probability of large vs small angle Coulomb scattering of the reacting nuclei to enable subsequent nuclear reactions via tunneling.
Aspects of screening effects to enable calculation of nuclear reaction rates are also evaluated, including Coulomb scattering and localized heating of the cold fuel, primary D-D reactions, and subsequent reactions with both the fuel and the lattice nuclei. The effect of screening for enhancement of the total nuclear reaction rate is a function of multiple parameters including fuel temperature and the relative scattering probability between the fuel and lattice metal nuclei. Screening also significantly increases the probability of interaction between hot fuel and lattice nuclei increasing the likelihood of Oppenheimer-Phillips processes opening a potential route to reaction multiplication. We demonstrate that the screened Coulomb potential of the target ion is determined by the nonlinear Vlasov potential and not by the Debye potential. In general, the effect of screening becomes important at low kinetic energy of the projectile.
Physical Review C – Novel nuclear reactions observed in bremsstrahlung-irradiated deuterated metals
d-D nuclear fusion events were observed in an electron-screened, deuterated metal lattice by reacting cold deuterons with hot deuterons produced by elastically scattered neutrons originating from bremsstrahlung photodissociation. Exposure of deuterated materials (Er D3 ad Ti D2) to photon energies in the range of 2.5–2.9 MeV resulted in photodissociation neutrons that were below 400 keV and also the 2.45-MeV neutrons consistent with 2H(d,n) 3 He fusion.
Additionally, neutron energies of approximately 4 and 5 MeV for TiD2 and ErD3 were measured, consistent with either boosted neutrons from kinetically heated deuterons or Oppenheimer-Phillips stripping reactions in the highly screened environment. Neutron spectroscopy was conducted using calibrated lead-shielded liquid (EJ-309) and plastic (stilbene) scintillator detectors. The data support the theoretical analysis in a companion paper, predicting fusion reactions and subsequent reactions in the highly screened environment.
SOURCES- NASA, Physical Review C
Written By Brian Wang, Nextbigfuture.com
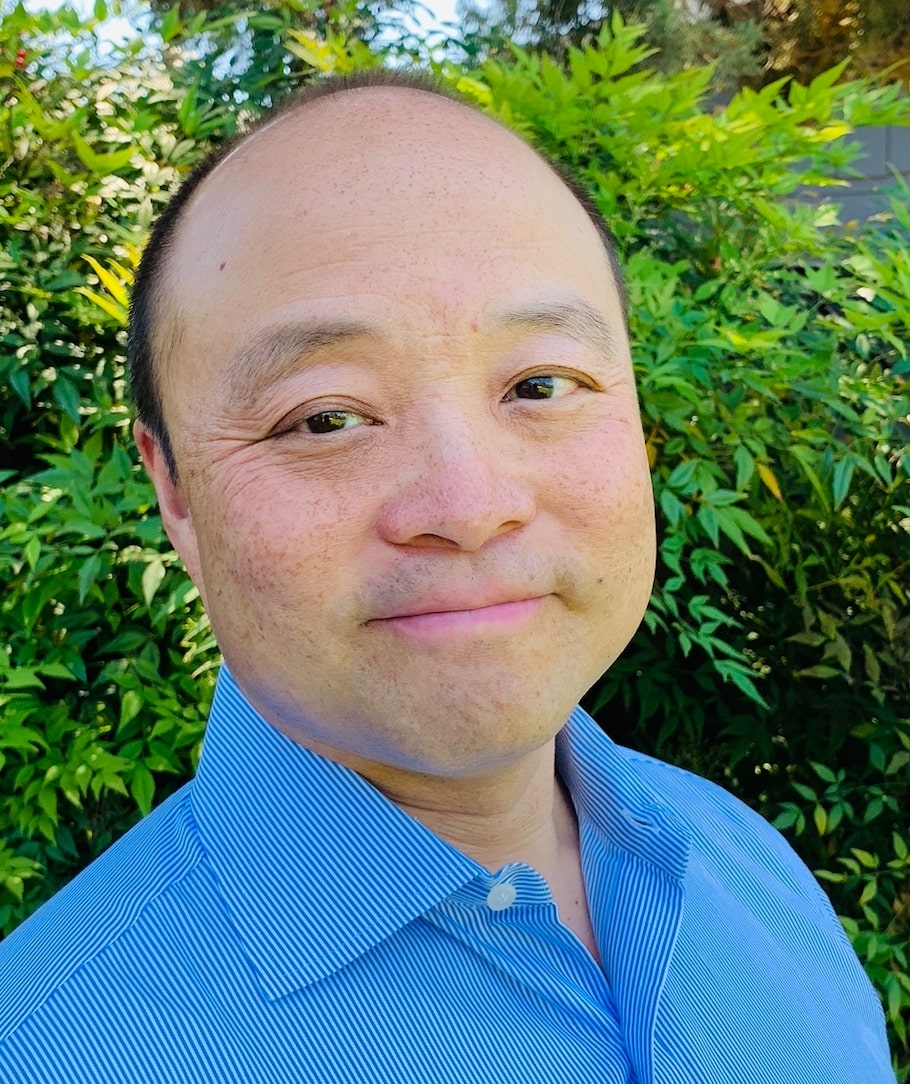
Brian Wang is a Futurist Thought Leader and a popular Science blogger with 1 million readers per month. His blog Nextbigfuture.com is ranked #1 Science News Blog. It covers many disruptive technology and trends including Space, Robotics, Artificial Intelligence, Medicine, Anti-aging Biotechnology, and Nanotechnology.
Known for identifying cutting edge technologies, he is currently a Co-Founder of a startup and fundraiser for high potential early-stage companies. He is the Head of Research for Allocations for deep technology investments and an Angel Investor at Space Angels.
A frequent speaker at corporations, he has been a TEDx speaker, a Singularity University speaker and guest at numerous interviews for radio and podcasts. He is open to public speaking and advising engagements.
Besides, you can’t very well get power from something like NIF anyway.
Now, self-eating fuel rods, perhaps with a skin tight layer of hard to fuse iron as a coating, maybe you could get a head of steam out of that, Tsander style—solid rocket fusion.
Oh, I'm personally convinced there is something going on with cold fusion.
It's just not understood enough to make reproduction predictable.
But given the actual statistics of replication, it is definitely a thing.
Calling Mary Yugo.
Mary Yugo, your presence is requested on the forum.
Are you sure that cold fusion deserves to get more credence?
Where Was The Excess Heat Coming From? ZPE?
Rats. Here I was hoping we had some cold fusion to power our EM drives.
For NASA's original purposes, space missions, we can probably discount the original fuel manufacture as something that can be done back on planet Dirt with cheap (coal?) power.
But yes, what we are REALLY interested in is the entire fuel cycle, energy balance, from a cubic metre of raw sea water through to the household power socket.
If this reaction is fusion and a neutron emitter, it would most likely need to be contained and treated as a source of radioactive byproducts.
So no big difference with nukes indeed.
This might work if the effect was obtained with just a thin layer on the surface of the reactants, and the reactive material was allowed to slowly erode.
Which may be the case here, if the reaction happens on the surface while irradiating the metal with an external source of gamma rays.
So if this electronic shielding and inter-atomic spacing (to enable tunneling) is the real gimmick, then are there any further such electronic gimmicks which can be looked into?
MCF doesn't reach breakeven, although it held one of the records for getting closest to it. The problem is alpha-sticking, whereby the alpha by-products poison the muon catalyst.
Some people say it's purely quantum tunneling – again, perhaps making use of the electronic shielding to allow closer proximity
Yes, I guess that's the angle. As was pointed out by Brett (or you?), the real trick is to see if there is power multiplication above 1.0. All in: cost (in energy) to make and probably 're-make' the deuteride, energy to make the pulsed gamma beam, energy to extract the ablative daughter products for the first step. I kind of like this article's approach though.
⋅-⋅-⋅ Just saying, ⋅-⋅-⋅
⋅-=≡ GoatGuy ✓ ≡=-⋅
This cannot work at high temperatures, so energy gain per volume must be low.
I posted a bit quicker, but you beat me on quality.
And ninja'd by Mike.
Based on my superficial understanding of the paper, they think that a big part of the process is that the deuterium ions are embedded in the electron clouds of the metals so they are shielded from each other's positive charge until they get very close.
Pure compressed gas doesn't give you that.
Otherwise you could make a nuclear piston engine, with the gas compressed by the piston to a critical density, the spark plug… err gamma ray generator gives you ignition, and your piston gets a power stroke.
As I understood, a key feature here is the charge masking provided by the metal's electrons. That makes the coulomb barrier easier to overcome. You won't have that in compressed deuterium gas.
I suspect that, if this work replicates, hundreds of people will get getting deuterated every compound they can, and zapping them with gamma rays to see what happens.
Fusion in deuterated materials is not new:
https://www.tandfonline.com/doi/abs/10.13182/FST92-A30100
A lot of fusion methods are nowhere close to gain without tremendous efforts, and yet it's still a respected field. I see no reason why it should be different with this. As long as a reaction is actually happening and is able to be reproduced, it should have scientific credence.
Materials physics is one of the fastest changing and growing fields in the sciences. There's a ton of things we've discovered in just the last decade and tons of things left to be discovered.
The evidence seems to be growing more and more that some type of event, possibly of nuclear origin, is happening in loaded metal lattices that can produce an anomalous heat effect. If this type of reaction is actually happening, I think it's only a matter of time before it becomes reproducible and has a ton of scientific merit. I don't think Fleischman and Pons and the field itself deserves the amount of disrespect it has gotten to date.
So… wait a second.
{atom}-{deuteride}ₓ gets the deuteroes 'close' to each other; in the words of the article, far closer than the inter-atomic spacing in a plasma setup, be it tokamak or Farnsworth Fusor or wiffle ball (magneto-electrostatic confinement), stellarator, or any of the 'hot' alternatives. The kinetic energy-to-fuze, AKA "heat" comes from a burst of gamma, which sends the deuterium ions zinging off at many keV of kinetic energy. Which in turn overcomes the coulomb + charge barrier, allowing them to fuze. Whoop-ee!
I got to thinking, but what about a 1000 bar pressurized gas? Not even close to really-high-pressure industrially, and certainly packing a LOT of deuterium atoms in a confined bottle. How many? Well, if an Avogadro's Number of atoms is a mole, and at ambient temperatures and pressures, it takes 22.4 liters of gas to make a mole, at 1000 bar, it only takes 22.4 milliliters. Avogadro's Big Number is 6.022×10²³, so that divided by 0.0224 liters is 2.69×10²⁵ molecules per liter.
That's pretty good. Let's see … put that under a 1, and you get 3.7×10⁻²⁶ liters per molecule. Hmmm.. liters are cubic decimeters or ¹⁄₁₀₀₀ m³, so hmmm… 3.7×10⁻²⁹ cubic meters. Take the cube root of that and we have 3.34×10⁻¹⁰ m on the side of each molecule's box. Which would be 0.334 nanometers on a side.
Last I checked, that'd be on the order of (or a bit less than) the packing of atoms in ionic solids such as Erbium Deuteride.
Yah know? G G
Well we still don't know exactly what pons and Fleishmann were doing but"cold fusion" was a terrible name for it. Muon catalyzed fusion is real and totally different.
Cold for physics is 600C which is a nice temperature for a GE stream turbine. That's where this is headed.
I don't think a crystal lattice is required, as long as the deuterons are close enough to each other. Liquid is still close to solid density, and in something like heavy water, there are two deuterons on each oxygen atom. That may be enough.
On 2nd thought, if heavy water worked, we should see similar effects in heavy water fission reactors. Yet AFAIK we don't. So either you're correct that it needs a lattice, or more likely, the oxygen doesn't enough masking. Or there may be some other factors.
Another interesting option is deuterated ammonia (ND3), which can be produced with the Haber process using deuterium in place of regular hydrogen. It can be kept liquid at elevated pressure. The nitrogen won't give any more masking than an oxygen, but it can form free electron solutions with alkali metals. Maybe that would work…
Yes, neutron production is an important little detail that differentiates this from other (aneutronic) LENR claims.
It may be a different reaction, maybe not. Until this is replicated and verified we'll know if there is actual nuclear fusion or not. Therefore and for the moment being, I'm placing it in the same bag of claimed reactions of deuterium in a metallic lattice.
I think that holding the deuterium very close to eachother in the crystal lattice is fairly critical. The crystal lattice doesn't exist in a liquid, and may change drastically at different temperatures depending on the materials.
1000C is more than you want most of the time.
For our standard big power production, we just want steam boiler temperature. For coal plants we run at 6-700C. Higher would be more efficient, but boiler materials don't last.
For nuke plants, they run a lot lower, substituting lower efficiency (because fuel is near free) for more reliability and less maintenance (which is much more expensive on the nuke).
I suspect a fusion plant would be much like an ordinary nuke in this respect.
Metals outgassing hydrogen is, AFAIK, just standard solution behaviour. So it'll only outgas to the extent that the external pressure of hydrogen is lower than the internal pressure of hydrogen.
Increasing the temperature increases the internal pressure, but if you increase the external pressure too then it should remain in balance.
What was that story about something that only worked when a random burst of gamma rays happened to …
Oh yeah, that's The Incredible Hulk origin story.
They were doing this
— with different materials
— without adding high energy x-ray/gamma rays to the metals, which NASA claim are the critical source of localized energy (unless there WAS some source of x-ray that P&F didn't know about)
— without neutron production, which was always the big red flag that made conventional nuclear physicists think that cold fusion didn't happen
Isn’t This What Pons & Fleischmanns were doing?
Sorta, but unlike LENR this produces neutrons and has a way of accelerating deuterons to the necessary kinetic energy.
I'm neutral on LENR but most LENR people don't actually think it's fusion anymore. If it works it's likely an entirely different process, maybe involving the weak force.
There's probably no requirement for the material to be solid, though. And I wonder how many electron shells are needed for the masking effects described here.
If light elements work, plain heavy water could be an option. If it evaporates – just run the steam through a turbine, and condense it back.
There was recently an article on NBF about some special arrangement where protons in a solid (a hydride maybe? I don't remember) acted like the electrons in a metal.
Something like that could possibly be done with deuterons, and that kind of bonding should be strong enough to keep them from outgassing. But I don't know any such arrangement with a high melting point. All the simple metal hydrides that I checked have fairly low melting points, and most of them don't act like this, AFAIK.
This is the "cold fusion" think, which I have been convinced is real for 10 years now. The problem I see with it is that the fusion reactions generate the heat energy that immediately destroys the lattice, thus ending the reaction. I believe this is the reason why no one has been about to commercialize this technology.
I once had the idle thought that somebody should build a whole array of cold fusion cells, and monitor them in real time. Who knows, maybe you'd find they were only working while clouds of dark matter passed through them, or something like that.
But, yes, that does seem to be the issue if it works as it's advocates suggest: It's self terminating.
All you need to do is get the deuterium atoms close enough for quantum tunneling to happen. There may be quantum effects in certain materials that might make this happen. I am not a Solid State physicist so I don't know how it could be done but I wouldn't dismiss the idea as impossible without looking at it.
As I mention elsewhere: if this is a reliable way to create the reaction, even if the COP is not generator worthy, it would still be a big discovery.
Reproducible experiments would give much more credence to the whole cold fusion/LENR domain.
Minimum acceleration with quantized inertia ~ 2E-10 m/s^2.
What relevance does this have when the thermal jiggle and jostle of atoms in solid matters is ~2 km/s in random directions? Not to say anything about atoms hit by x-rays.
A recurrent problem of cold fusion experiments seems to be that the reaction itself changes the magic juju that allows it to start.
So it works in a flash, then nothing.
Or just nothing, given the magic incantations and planetary alignment required to make it work not always were there.
Cold fusion was ridden with lousy replication numbers since day 0.
If the NASA people found a way to make it work 100% of the time, then that's new.
I honestly don't think you could maintain high loading at elevated temperatures, the metal would outgas. But I could be wrong about that.
My guess is that if you want to reach meaningful power levels it will have to be done in something approaching bulk. But the text also says that you can load titanium with deuterium, i.e. a few hundred degrees should be possible.
Furthermore, if you coat the titanium slab with a micron of something really tough, you could come close to the melting point of titanium, i.e. above 1000 degrees Celsius.
My question is how you should produce the gamma rays effectively? If you have an a Q of 10 for the fission, you need at least 10% efficiency (electricity to gamma rays) just to break even when the energy of the gamma ray generation is considered.
Quantised inertia
If you're doing it in a foil, the foil could remain cold while a lot of power was leaving in the form of energetic particles, just because it didn't intercept most of them.
The question isn't whether it works. Fusors work, and you could build one in your basement.
The question is whether it produces a useful GAIN.
Then again, fusors actually see practical use as neutron sources for imaging, I suppose this could see the same use even if it didn't produce net power out.
Though there is nothing about energy out vs energy in. Just achieving fusion has been done in the lab for generations. It's getting it to pump out more power than it uses that's the tricky bit.
It does spring to mind that maybe those "cold fusion" tests that did suddenly, randomly, non-reproducibly, give a burst of excess heat might have been irradiated with some sort of x-ray?? Maybe from electric sparks from crappy connections?
Then when they try to replicate, they set the system up well, with good connections… nothing.
Calling Stanley Pons, calling Stanley Pons, calling Stanley Pons … you should have tried this. Rest in peace, Martin Fleischmann.
Now THIS looks promising.
Reason it can't work in 5, 4, 3…
Actually, if the metal target has to be cold that kind of makes it difficult to generate power. Though this may be physicist talk, where "cold" means "not a plasma".