A game-changing technique for imaging molecules known as cryo-electron microscopy has produced its sharpest pictures that for first-time can see individual atoms in a protein.
By achieving atomic resolution using cryogenic-electron microscopy (cryo-EM), researchers will be able to understand, in unprecedented detail, the workings of proteins that cannot easily be examined by other imaging techniques, such as X-ray crystallography.
Cryo-EM has been around for decades. It has long been able to determine the shape of flash-frozen samples by firing electrons at them and recording the resulting images. Advances in technology for detecting the ricocheting electrons and in image-analysis software catalyzed a ‘resolution revolution’ that started around 2013. This led to protein structures that were sharper than ever before — and nearly as good as those obtained from X-ray crystallography, an older technique that infers structures from diffraction patterns made by protein crystals when they are bombarded with X-rays.
The best resolution with Cryo-EM dropped from 9 angstroms in 2002 to 4 angstroms in 2008 to 2 angstroms in 2014 and now to 1.2 angstroms. An angstrom is 0.1 nanometers.
Different proteins have different stability. The best resolutions are reached on the most stable proteins like an iron-storing protein called apoferritin. The protein has become a testbed for cryo-EM. A resolution of 1.54 ångströms was the previous record and now it is 1.2 angstroms. Further improvements will push this to a limit with Cryo-EM of about 1.0 angstroms. Less stable proteins are currently at 1.7 to 2 angstroms. The researchers expect a further 20% resolution improvement.
They used an instrument that ensures that the electrons travel at about the same speed before hitting a sample which enhances the resolution of the resulting images. Scheres and team used a different technology to fire electrons traveling at similar speeds. They reduced the noise generated after some electrons careen off the protein sample and had a more sensitive electron-detecting camera. Their 1.2-ångström structure could pick detect individual hydrogen atoms, both in the protein and in surrounding water molecules. Combining all of the methods for reducing variance in electron speed and reducing noise will help them to get to about 1.0 angstrom resolution in the most stable protein images.
SOURCES- Nature
Written by Brian Wang, Nextbigfuture.com
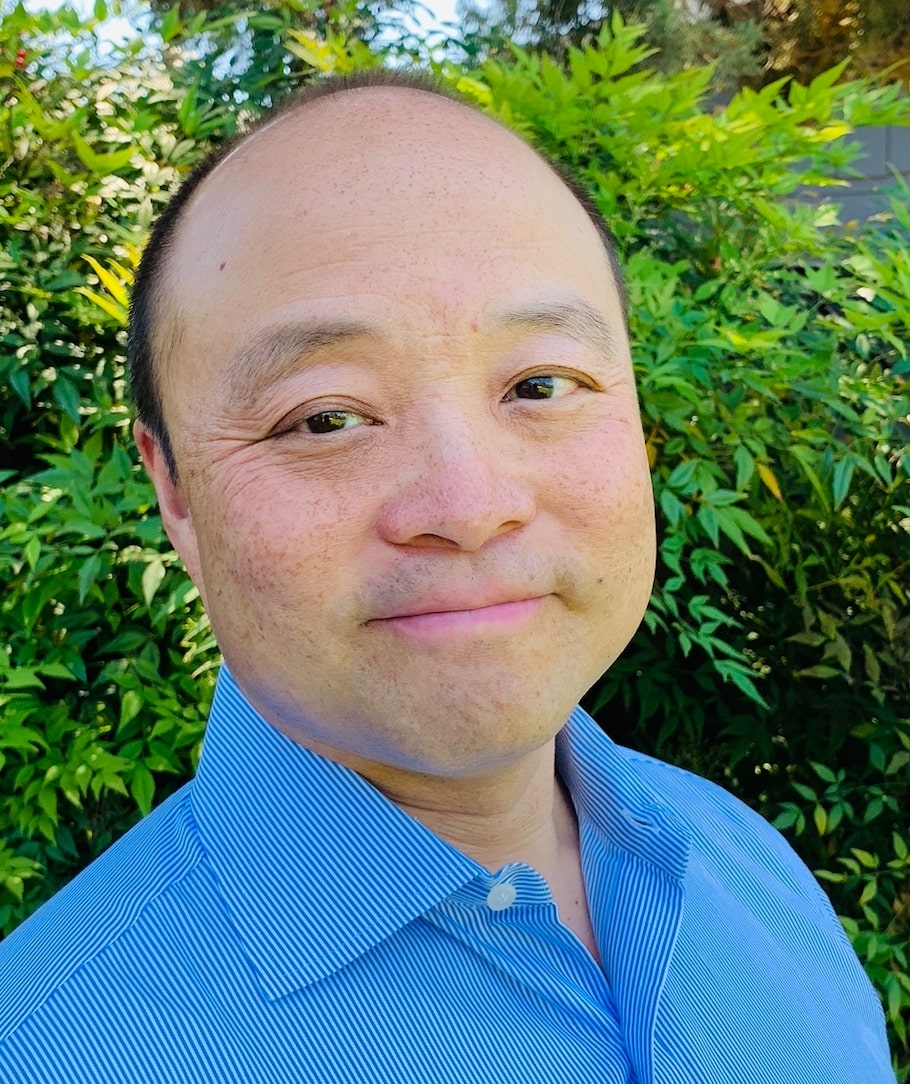
Brian Wang is a Futurist Thought Leader and a popular Science blogger with 1 million readers per month. His blog Nextbigfuture.com is ranked #1 Science News Blog. It covers many disruptive technology and trends including Space, Robotics, Artificial Intelligence, Medicine, Anti-aging Biotechnology, and Nanotechnology.
Known for identifying cutting edge technologies, he is currently a Co-Founder of a startup and fundraiser for high potential early-stage companies. He is the Head of Research for Allocations for deep technology investments and an Angel Investor at Space Angels.
A frequent speaker at corporations, he has been a TEDx speaker, a Singularity University speaker and guest at numerous interviews for radio and podcasts. He is open to public speaking and advising engagements.
No water in AlphaFold. In fact, not in any of the computational approaches because water is really special like it is everywhere in biological tissues that the guys just ignore.
Well this is potentially much better than the protein folding stuff. At least we have water in the picture albeit under non physiologic conditions. The protein folding stuff needs to incorporate water into its computation models to be believable.
The AI can predict, it can't measure. By taking the results from this method the AI can be refined to make more accurate predictions. Modelling by itself is never sufficient, models need to be continuously refined by running against independently obtained results.
"Solved" is an overstatement. They can predict a theoretical structure, which matches experimental results in some cases (still far from all cases, AFAIK), within some small margin of error.
Here we are talking about experimental measurement of the structure, which is a separate problem. And then there is function, which is another problem altogether.
Currently they can determine protein structure experimentally with X-Ray crystallography, but it's like having a CT or an MRI – different techniques give you different info.
Isn't it already solved by AlphaFold AI?
It's either 20 billion FPS or cryo, not both. Once you freeze things, they stop moving. Which means this particular technique can only give structural info of likely deformed proteins (deformed by the cold). It can't observe their function in a live environment.
That said, if they flash-freeze live samples, they get sort of a snapshot of what the proteins were doing when they were frozen. Do that a lot, and they can somewhat reconstruct the function.
OK, not bad, but not quite there. A hydrogen atom is about 1.2 Angstrom, so they can just about resole one hydrogen atom. If they would reach 0.1 Angstrom, I think that the problem would be considered "solved". I suppose you would need that kind of resoution to be able to judge the function of different atoms in proteins..
… one that can work underwater.
Unfortunately, what they probably need is a 20 billion FPS cryo-EM camcorder.