Our current space telescopes have found thousands of exoplanets based upon the slight variation in movement that they cause to the stars they are orbiting, but we have not seen the reflected light from those exoplanets.
NASA has a count of exoplanets and candidate exoplanets.
We need better and larger space telescopes so that we can see and analyze the reflected light of the atmospheres of those exoplanets. The spectrum of the reflected light from any exoplanet with atmospheres will tell us if there is an atmosphere and what gases are in those atmospheres. We would be looking for the same mix of gases that we have in our atmosphere. Our atmosphere only exists as it does because of life on Earth. If oxygen is detected in an atmosphere then this is a good indication that there is life. There are also trace elements that would indicate technological civilizations.
However, the James Webb Space telescope might only be able to check 1 to 3 of the habitable zone exoplanet atmospheres.
An Array of 35, 8.5 meter lens space with an inflatable structure could be launched with two SpaceX Super Heavy Launches and form the equivalent of a 50 meter space telescope. This would be able to scan a thousand or more exoplanet atmospheres for signs of live.
The information that we would get would just be the general composition of the atmosphere of the planets. If we got the info about 1-3 exoplanets, it would be interesting but we would not have enough data to compare what was happening. It we got a thousand atmosphere readings and the different orbits, then we would have more information to analyze and determine more about what was happening.
The Nautilus space telescope is a system that could get launched by 2030 and operating by 2033. It would be affordable and have 50 times the light collection area of the James Webb Telescope.
It would be lightweight and affordable.
They would replace Primary Mirrors with Ultralight Diffractive Lenses: Nautilus will use an 8.5 meter diameter multi-order diffractive engineered material lens (MODE lens) instead of a primary mirror. These diffractive lenses have ∼100× lower areal density than traditional mirrors, are 100–1,000× less sensitive to misalignments (drastically reducing fabrication and integration costs), and can be cost-effectively replicated (via pressure molding). MODE lenses have been recently developed by our team: these lenses are enabled by revolutionary progress in optical free-form fabrication and high-fidelity pressure molding of low-temperature optical glasses.
Proving this technology would enable even larger and lighter future space telescope arrays.
Mission Architecture: Nautilus will be launched in a compact configuration to minimize launch volume. Once in orbit the telescope will use an inflatable balloon to deploy the MODE lens and lock-in struts will be used to stabilize the lens at its nominal position. Focusing and alignment of the instrument will be achieved through a tip-tilt and focusing mechanism in each arm (visual and near-infrared) of the instrument. Power will be provided by a flexible solar cell film with space heritage. High-precision pointing will be provided by reaction wheels. Thrusters will be used to offload the accumulated angular momentum.
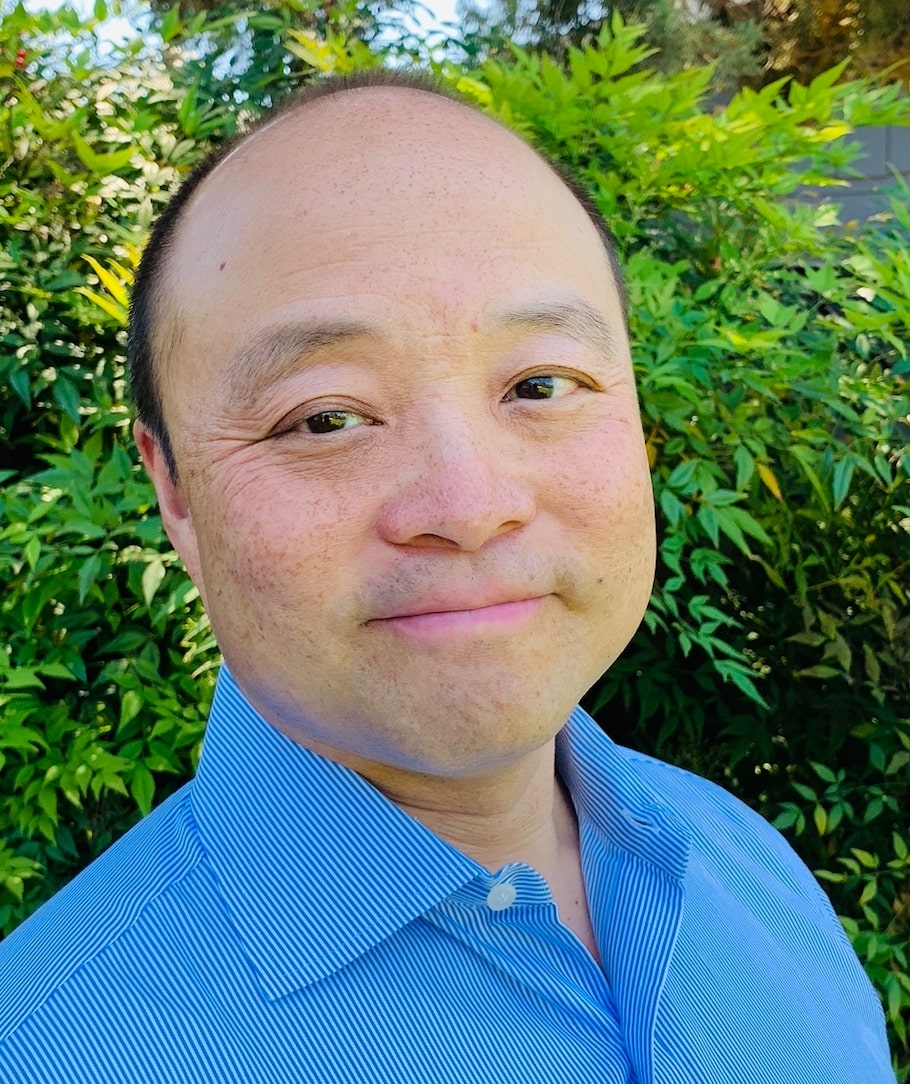
Brian Wang is a Futurist Thought Leader and a popular Science blogger with 1 million readers per month. His blog Nextbigfuture.com is ranked #1 Science News Blog. It covers many disruptive technology and trends including Space, Robotics, Artificial Intelligence, Medicine, Anti-aging Biotechnology, and Nanotechnology.
Known for identifying cutting edge technologies, he is currently a Co-Founder of a startup and fundraiser for high potential early-stage companies. He is the Head of Research for Allocations for deep technology investments and an Angel Investor at Space Angels.
A frequent speaker at corporations, he has been a TEDx speaker, a Singularity University speaker and guest at numerous interviews for radio and podcasts. He is open to public speaking and advising engagements.
Very nice and versatile solution for a space telescope.
A good fit to place at 545 AU orbit too when using the sun as a gravitational lens. As easily transported in a smaller package.
Although I wonder if local materials from objects in the Kuiper Belt could used for station keeping reaction mass and some basic materials to support the structure etc.
If possible this aiaa analysis from February this year may be relevant:
https://arc.aiaa.org/doi/10.2514/1.A35493
How big does it have to be to see well defined land masses, bodies of water, glaciers and vegetation on exo-planets?
REALLY Big, in general.
There is a fairly well known equation known as the Raleigh Angular Resolution limit equation, that actually answers your question mathematically. ( https:\\en.Wikipedia.org\wiki\Angular_resolution )
In short the imaging problem depends on how far away the planet we’re spying is located at. If it is at one of our very closest neighbors (within 10 light years), well that’s one thing. If it is at 100 ly or 1000 … that’s 10× to 100× more distant, and requires a telescope 10× to 100× bigger just to see the same continents, glaciers and oceans. Or other things…
But look at the ‘within 10 LY’ or easiest to directly image case. Further, Léts say that the smallest thing we are willing to accept at the limits of our purported scope are 1,000 km in size. Smaller than any of Earth’s continents, so one might get actual pixels (plural) of resolution, defining the shape of the continents and oceans instead of just single dots.
In kilometers, 10 light years is
10 years × 365 days × 24 hours × 60 minutes × 60 seconds × 299,452 km / second
= 94,608,000,000,000 km
That’s our baseline to the star. Angular ‘resolution’ is very close to “thing size / baseline”, so
1,000 km ÷ 94,608,000,000,000
= 0.000000000106 radians (units of angular resolution)
Then, using Raleigh’s formula
ar (radians) = 1.22 • wavelength / diameter (in meters)
0.000000000106 = 1.22 × 0.00000550 (green light) / diameter, so rearrange
diameter = 1.22 × 0.00000550 ÷ 0.000000000106
diameter = 635,000 meters
diameter = 635 kilometers
diameter = 400 miles.
SO. A mirror with a diameter of 400 miles or 635 km. Like the first words … REALLY BIG.
________________________________________
For my occasional readers, it also should be noted (as per other comments) that positioning a much, much smaller telescope at the gravitational ‘focal point’ of the Sun (about 500± AU away) would serve to do 3 very important things: multiply the brightness of the exoplanet by billions of times, multiply its apparent angular distance from its parent star by 100,000 times, and thus reduce the size of the telescope by the same 100,000 (to a few million) times. Thus, bringing it into the size of this article’s Fresnel-type refraction-ring lens concepts.
See? Physics (and without rancor … much math) is good. It predicts things!!!
⋅-⋅-⋅ Just saying, ⋅-⋅-⋅
⋅-=≡ GoatGuy ✓ ≡=-⋅
Providing a link to the telescope proposers site might have been helpful…
https://nautilus-array.space/