Over the next two decades China hopes to build the world’s largest nuclear power industry. Plans include as many as 30 new conventional nuclear plants (in addition to the 34 reactors operating today) as well as a variety of next-generation reactors, including thorium molten-salt reactors, high-temperature gas-cooled reactors (which, like molten-salt reactors, are both highly efficient and inherently safe), and sodium-cooled fast reactors (which can consume spent fuel from conventional reactors to make electricity). Chinese planners want not only to dramatically expand the country’s domestic nuclear capacity but also to become the world’s leading supplier of nuclear reactors and components.
The Shanghai Institute’s effort to develop molten-salt reactors, a technology that has sat all but forgotten in the United States for decades, reflects just how daring China’s nuclear ambitions are. Already, the government has invested some two billion Chinese renminbi ($300 million) over the last five years in molten-salt R&D. Building actual plants will require tens of billions more. As with other innovative nuclear technologies in development around the world, there are few guarantees: though people have run small, experimental molten-salt reactors, no one’s ever actually built one at utility scale and hooked it up to the grid. Yet the Chinese government expects to have a commercial-size plant up and running within 15 years, helping to revive the beleaguered nuclear power industry.
The China Academy of Sciences in January 2011 launched an R&D program on LFTR, known there as the thorium-breeding molten-salt reactor (Th-MSR or TMSR), and claimed to have the world’s largest national effort on it, hoping to obtain full intellectual property rights on the technology. The TMSR Research Centre has a 5 MWe MSR prototype under construction at Shanghai Institute of Applied Physics (SINAP, under the Academy) originally with 2015 target for operation, now 2020. SINAP has two streams of TMSR development – solid fuel (TRISO in pebbles or prisms/blocks) with once-through fuel cycle, and liquid fuel (dissolved in FLiBe coolant) with reprocessing and recycle. A third stream of fast reactors to consume actinides from LWRs is planned.
The TMSR-SF stream has only partial utilization of thorium, relying on some breeding as with U-238, and needing fissile uranium input as well. SINAP aims at a 2 MW pilot plant (TMSR-SF1) initially, and a 100 MWt experimental pebble bed plant (TMSR-SF2) with open fuel cycle by about 2025, then a 1 GW demonstration plant (TMSR-SF3) by 2030. TRISO particles will be with both low-enriched uranium and thorium, separately.
The TMSR-LF stream claims full closed Th-U fuel cycle with breeding of U-233 and much better sustainability with thorium but greater technical difficulty. SINAP aims for a 2 MWt pilot plant (TMSR-LF1) by 2018, a 10 MWt experimental reactor (TMSR-LF2) by 2025 and a 100 MWt demonstration plant (TMSR-LF3) with full electrometallurgical reprocessing by 2035, followed by 1 a GW demonstration plant.
A TMSFR-LF fast reactor optimized for burning minor actinides is to follow.
SINAP sees molten salt fuel being superior to the TRISO fuel in effectively unlimited burn-up, less waste, and lower fabricating cost, but achieving lower temperatures (600°C+) than the TRISO fuel reactors (1200°C+). Near-term goals include preparing nuclear-grade ThF4 and ThO2 and testing them in a MSR. The US Department of Energy is collaborating with the China Academy of Sciences on the program, which had a start-up budget of $350 million. The target date for TMSR commercial deployment is 2032.
Other molten salt project
Canada-based Terrestrial Energy Inc (TEI) has designed the Integral MSR. This simplified MSR integrates the primary reactor components, including primary heat exchangers to secondary clean salt circuit, in a sealed and replaceable core vessel that has a projected life of seven years. The IMSR will operate at 600-700°C, which can support many industrial process heat applications. It operates in the thermal neutron spectrum with a hexagonal arrangement of graphite elements forming the moderator. The fuel-salt is a eutectic of low-enriched (2-4%) uranium-235 fuel (as UF4) and a fluoride carrier salt – likely sodium rubidium fluoride with potential to change to FLiBe – at atmospheric pressure. Secondary loop coolant salt is ZrF4-KF. Multiple pumps and six heat exchangers allow for redundancy. Emergency cooling and residual heat removal are passive. When the sealed core is replaced after seven years, it is then left for fission products to decay. Each plant would have space for two reactors, allowing seven-year changeover, with the used unit removed for off-site reprocessing when it has cooled.
The IMSR is designed in three sizes: 80 MWth (32.5 MWe), 300 MWth, and 600 MWth. The total levelized cost of electricity from the largest is projected to be competitive with natural gas.
he smallest is designed for off-grid, remote power applications, and as a prototype. The company expects to complete CNSC pre-licence review by the end of 2016, and hopes to commission its first commercial reactor by the early 2020s. In January 2015 the company announced a collaborative agreement with US Oak Ridge National Laboratory (ORNL) to advance the design
SOURCES – World nuclear association, MIT Technology review
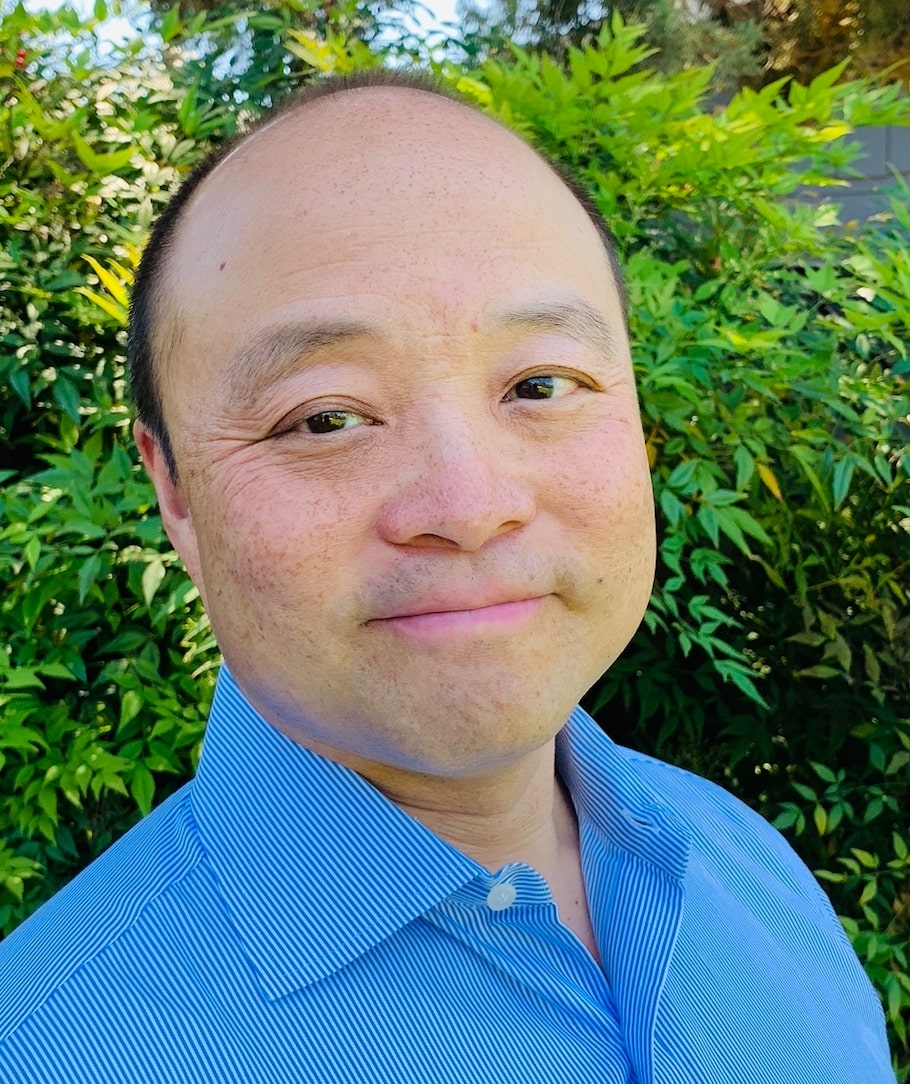
Brian Wang is a Futurist Thought Leader and a popular Science blogger with 1 million readers per month. His blog Nextbigfuture.com is ranked #1 Science News Blog. It covers many disruptive technology and trends including Space, Robotics, Artificial Intelligence, Medicine, Anti-aging Biotechnology, and Nanotechnology.
Known for identifying cutting edge technologies, he is currently a Co-Founder of a startup and fundraiser for high potential early-stage companies. He is the Head of Research for Allocations for deep technology investments and an Angel Investor at Space Angels.
A frequent speaker at corporations, he has been a TEDx speaker, a Singularity University speaker and guest at numerous interviews for radio and podcasts. He is open to public speaking and advising engagements.
Comments are closed.