Nextbigfuture wrote this material which has been made in a chinese lab. The material is 20 times stronger than Kevlar.
The material would enable flywheel energy storage with jet engine or rocket like energy density.
Adam Crowl indicates super flywheels would be better than rocket fuel when oxygen is factored
For carbon nanotube material, with a density of about 1,800 kg/m3, and an operating maximum stress of 54 GPa, that means a specific energy density of 7.5 MJ/kg. For comparison a kilogram of natural gas represents about 50 MJ of chemical energy, if fully burned. However that comparison neglects the efficiency that the chemical energy can be turned into useful work. Flywheels convert their stored energy into electricity at very high efficiency – 95% or so. Running a gas powered generator turns maybe 20% of the gas’s chemical energy into electrical energy. The rest is lost as heat, via both friction and the Carnot Limit. That’s still 10 MJ/kg, so why use a flywheel? The other assumption is that one can neglect the mass of the oxygen in the air that burns the fuel. If the application we’re using the flywheel for is in space, then the oxygen has to be added in.
Every molecule of methane masses 16 atomic mass units (16 amu), while every molecule of oxygen (O2) masses 32 amu. For every kilogram of methane burned, one needs 4 kilograms of oxygen to burn it with. Thus the useful energy content is 20% of the 10 MJ/kg we calculated above. In that case flywheel storage is better than chemical energy.
Goatguy calculated the flywheel explosive failure potential
Anyway, I learned that a material with a ρ (density) of 1.5 kg/L (i.e. about that of composite CWNT) if in ‘rod’ form, has a performance of about 8,100 MPa/kWh. Makes no difference the diameter, or the length of a particular fiber, when a balanced fiber is spun around its midpoint, the “performance” of the material is about 8,100 megapascals per kilowatt hour of kinetic rotational energy.
So, if the maximum working tensile strength of something like Kevlar is about 2,000 MPa, then one quite easily can reverse that and say: 2,000 ÷ 8,100 → 0.25 kWh per kilogram of Kevlar.
Now this article is claiming that the long-nanotube bulk Chinese material is “20× stronger” than Kevlar, or a working strength of 40,000 MPa. Easy enough, it ought to then store 20× the kinetic energy per kilogram as a performance metric. 40,000 ÷ 8,100 → 5 kWh/kg.
While that’s not exactly 10.6 kWh/kg (per some of the summary calculations of the referenced article), that’s OK. Its within shooting distance. Maybe “breaking strength” versus “working strength?” Sure.
More importantly, are the Glib Gotchas.
The first Glib Gotcha is that, well, a real, working kinetic energy flywheel would have to have… an enclosure, bearings, magnetic hoo-ha to invest and return energy to the flywheel. It’d need a safety shroud, gimbals (think of the inertial moment! of gyroscope effect) and metrology to figure out how it is working.
More mass, that.
Then there is “when it accidentally blows apart like a BOMB”, the maker has to balance the mass (and contained energy) of the flywheel with the enclosure’s ability to handle that energy, all turned … inevitably… into shock forces and HEAT.
For instance, if 100 kg of CWNT stuff is the storage medium, at 5 kWh/kg, it’d hold about 500 kWh of kinetic energy. Blowing itself to bits because of some structural problem results in 500 kWh × 3.6×10⁶ J/kWh → 1.8×10⁹ joules of thermal energy being released in a few milliseconds.
Putting THAT into perspective, 1.8×10⁹ J ÷ 4,186 J/g (TNT) → 430 kg of TNT.
Its also rotating over 250,000 RPM.
Tell me, what kind of container is going to be needed to contain a HALF TON of TNT going off all at once?
Goatguy analysis
REALLY good electrical-to-thermal conversion of a liquid to a high temperature expanding gas, using liquid argon for its cheapness, compactness and so on, wanting say an ISP of 500 ( × 9.81 = 4,900 m/s out-the-exhaust speed), each kg of exhaust has ½mv² → ½(1 × 4900²) → 12 MJ of kinetic energy. It also has a specific heat of 0.5 kJ/kg-K, and our plasma needs to be over 4,000° K heated. So I’ve heard. So, that becomes 2 MJ/kg for plasma heating.
14 MJ/kg. Our thruster’s flywheels deliver 14.4 MJ/kg. So… that means that each kg of argon requires a kilogram of flywheel more or less.
The thrust of each kilogram is 4900 newton-seconds. So, the thrust of (1 ⊕ 1 = 2) kg of argon and flywheel becomes 4900 ÷ 2 → 2450 newton-seconds per overall kilogram.
You really would want to eject the dead flywheel cores, continuously. Its a terrible ratio.
Supposing that you have a pretty sizeable (as in the size of the illustration) electric-gas powered 3rd stage (are we agreed?) that weighs in at oh, 25,000 kg, and is 70% reaction-mass-plus-flywheels (≡ 17,500 kg at a ratio of 0.97 flywheel-to-reaction mass), well let’s see.
Using Tsiolkovsky’s Rocket Equation, and NOT ejecting the flywheels (i.e. using them in parallel for maximum thrust), our rocket weighs 25,000 kg to start, and 16,136 at end. It has used up 8,864 kg of reaction mass, and accelerated it at 100% efficiency to 4900 m/s (ISP = 500). The ΔV is 2,150 m/s.
Using the SAME mass — 17,500 kg or 70% of 25,000, but as one of 3 binary fuel options (CH₄ + O₂, H₂ + O₂, Kerolox + O₂), with their posted ISP’s of 350, 450 and 320 respectively, And using them up, the ΔV at end-of-burn is respectively 4,130, 5,300 and 3,850 m/s.
See what I mean?
The flywheel-in-parallel thing while it does well for concentrating thrust and power, it leaves so much dead weight around that it isn’t competitive. Even at 6 kWh/kg of the flywheel nanotube stuff.
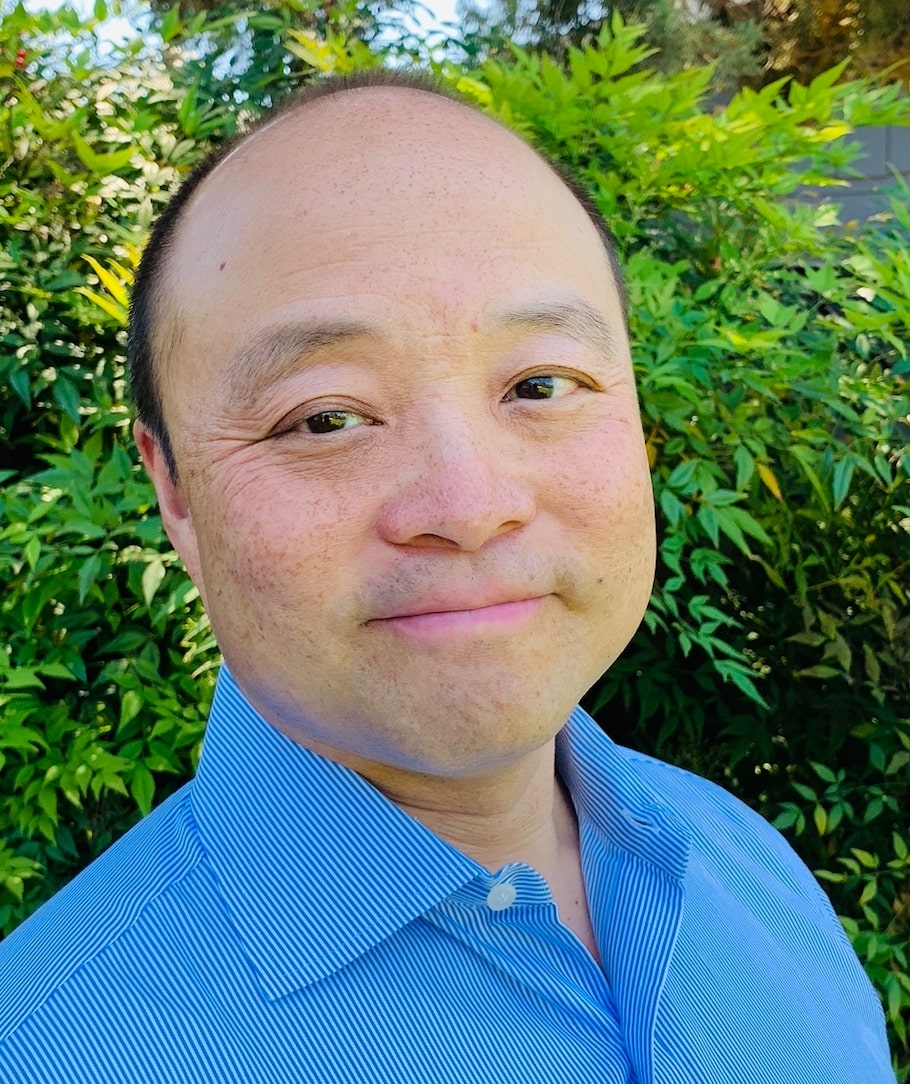
Brian Wang is a Futurist Thought Leader and a popular Science blogger with 1 million readers per month. His blog Nextbigfuture.com is ranked #1 Science News Blog. It covers many disruptive technology and trends including Space, Robotics, Artificial Intelligence, Medicine, Anti-aging Biotechnology, and Nanotechnology.
Known for identifying cutting edge technologies, he is currently a Co-Founder of a startup and fundraiser for high potential early-stage companies. He is the Head of Research for Allocations for deep technology investments and an Angel Investor at Space Angels.
A frequent speaker at corporations, he has been a TEDx speaker, a Singularity University speaker and guest at numerous interviews for radio and podcasts. He is open to public speaking and advising engagements.
A 10 kWh/kg flywheel should be safe when installed several metres below ground level. Are there other economical safe containments?
So flywheels could be the next superbattery, but still not enough to have battery powered rockets.They still allow much better battery powered vehicles. Especially for the users who are brave enough to take off the “deadweight” shatter shields around the flywheel and run them “naked” and 500 kg lighter.Who remembers the cookie cutter nanobots from The Diamond Age?
Ah, if you’ve got this high energy flywheel, couldn’t you ablate the rim at just the right point to provide controlled thrust? Providing relatively direct transformation of stored energy into thrust, and lowering the mass of the vehicle as the flywheels were exhausted?
Lots of people are working on carbon nanotube materials, China has no exclusivity, and it is not destined to control the world as many Chinese people believe from the simple reason that other nations do not want to be controlled by oppressive China.
not sure how to drive a rocket with a flyweel, but if it is possible, then there is also the option to just take the risk of it to explode (a havier launchpad), in worst case, it just wont lift off.It might be more interesting to use it for an (cyclic) electric railgun to space
Based on GoatGuy’s calculus, he concluded in the previous articles that a cylindrical shape is the wrong shape for a flywheel. But every flywheel article I’ve found suggests the opposite. There’s even work on hubless flywheels with magnetic bearings, to eliminate the less useful weight and cost of the hub. You’d think that if that was the wrong shape, someone would’ve picked up on that.My own analysis supports the contrary conclusion that a cylindrical shell is the best shape. I’ve posted the full analysis in NBF /2018/10/cnt-bundle-material-for-flywheels-40-times-better-than-batteries html. In summary:Neglecting interaction between volume elements, for a volume element dV = dA*dr at distance r from the axis and angular velocity w, under pure radial load:Esp = 0.5*(w*r)^2 (specific energy, [J/kg])Ysp = Lsp = w^2*r*dr (specific radial load, directly comparable to specific yield strength, [N*m/kg])Esp/Ysp = 0.5*r/dr ([J/N*m] aka unitless)dr is constant, so for any given specific yield strength Ysp, the max specific energy is proportional to the distance from the axis, r.If we assume the fibers arranged in a circumferential loop, with the loads translated to tangential loads, then using the formula for hoop stress of a thin-walled cylinder, we get:Ysp = Lsp = (w*r)^2 (specific tangential load, directly comparable to specific yield strength, [N*m/kg])Esp/Ysp = 0.5 ([J/N*m] aka unitless)Under pure hoop load, both load and energy scale quadratically with the distance from the axis, so the max specific energy depends only on the specific yield strength, regardless of mass distribution.These results also fit Adam Crawl’s energy density value, if we allow for an extra factor of 2 for safety margin and housing:Y = 54 [GPa] = 5.4e10 [Pa]d = 1800 [kg/m^3]Ysp = Y/d = 3e7 [N*m/kg]Esp = 0.5*Ysp (my result) * 0.5 (safety and housing) = 0.5 * 0.5 * 3e7 [N*m/kg] = 7.5e6 [N*m/kg] = 7.5 [MJ/kg]Furthermore, GoatGuy’s result of 10.8 [L*MPa/kWh] doesn’t seem to match published performance figures (unless I calculated wrong). These results lead me to believe there is an error in GoatGuy’s analysis.
Makes me wonder if there would be a way to store energy by spiing individual nanotube/nanorods on their axis. Too lazy to do the math -_-
2500 MPa steel can cost less than $1/kg. This steel may be competitive to be a flywheel rotor material.
Like GoatGuy I doubt that a flywheel powered rocket is useful, but flywheels for storing power for when a satellite goes into the shadow of a planet, or for power for a moonbase at night it seems useful.For powering a vehicle on a planet with no free oxygen, it is probably the best option. If the axis is vertical a failure can have the debris directed away from occupants of the vehicle.
A flywheel should be a giant charged battery in and of itself, or a fuel cell–something that isn’t just dead weight–RTGs in a hoop—something.
A flywheel should be a giant charged battery in and of itself, or a fuel cell–something that isn’t just dead weight–RTGs in a hoop—something.
Same profile as a space elevator “cable” for the same basic reasons.
Same profile as a space elevator “cable” for the same basic reasons.
apple peeler rocket!
Goatguy pointed out that the cnt flywheel is dead weight after being exhausted. Something to consider is viewing the cnt as a bulk construction material for space based use. In effect, it’s kinda like a refuelling rocket burning its’ fuel to reach space, and still having the fuel when it gets there. The fuel only being an example of a cargo.
apple peeler rocket!
Goatguy pointed out that the cnt flywheel is dead weight after being exhausted.
Something to consider is viewing the cnt as a bulk construction material for space based use. In effect, it’s kinda like a refuelling rocket burning its’ fuel to reach space, and still having the fuel when it gets there. The fuel only being an example of a cargo.
Right, 2*ro [Pa/J] (more correctly, [Pa*kg/J]) for a cylindrical shell is indeed what you’d get from my result with a bit of algebra.For now, I’ll take your word on the 3*ro [Pa/J] for radial fibers, since I’m having trouble with the math. I’ll just note that 2.4/3.6 = 2/3, where 2.4 is the ratio you posted earlier, and 3.6 is the systemic error. So it seems our math now agrees. And of course, lower [Pa/J] is better.Where I’m stuck is trying to integrate the centrifugal forces to account for the pull of the outer layers. The centrifugal force F(x) on each volume element due to its own motion is F(x) = ro*dV*w^2*x (x being being the radial coordinate). When I try to integrate that over x from r to OD, I get 0.5*ro*dV*w^2*(OD^2 – r^2), which is in Joules, and not Newtons. I’m probably doing the wrong integral, but I’m not sure what the correct form is. Integrals were never my strong suit.
Here it s, 2:30 in the morning, and I’m having to eat Humble Pie. Grrr… turns out that the tension in a thin walled rectangular torus (cylindrical shell) “performance” reduces to 2ρ in terms of Pa/J tensile stress per unit kinetic energy stored.The long thin rod (i.e. “fiber”) is “performance” = 3ρ Pa/J. Humble pie.Mmmm, good a la mode.LOLGoatGuyPS: my mistake was systemic: the 3.6 kWh → MPa factor was creeping in silently to upend the calculus. I ••• hate it ••• when that happens.
Right, 2*ro [Pa/J] (more correctly, [Pa*kg/J]) for a cylindrical shell is indeed what you’d get from my result with a bit of algebra.
For now, I’ll take your word on the 3*ro [Pa/J] for radial fibers, since I’m having trouble with the math. I’ll just note that 2.4/3.6 = 2/3, where 2.4 is the ratio you posted earlier, and 3.6 is the systemic error. So it seems our math now agrees. And of course, lower [Pa/J] is better.
Where I’m stuck is trying to integrate the centrifugal forces to account for the pull of the outer layers. The centrifugal force F(x) on each volume element due to its own motion is F(x) = ro*dV*w^2*x (x being being the radial coordinate). When I try to integrate that over x from r to OD, I get 0.5*ro*dV*w^2*(OD^2 – r^2), which is in Joules, and not Newtons. I’m probably doing the wrong integral, but I’m not sure what the correct form is. Integrals were never my strong suit.
Here it s, 2:30 in the morning, and I’m having to eat Humble Pie.
Grrr… turns out that the tension in a thin walled rectangular torus (cylindrical shell) “performance” reduces to 2ρ in terms of Pa/J tensile stress per unit kinetic energy stored.
The long thin rod (i.e. “fiber”) is “performance” = 3ρ Pa/J.
Humble pie.
Mmmm, good a la mode.
LOL
GoatGuy
PS: my mistake was systemic: the 3.6 kWh → MPa factor was creeping in silently to upend the calculus. I ••• hate it ••• when that happens.
I neglected the tension-compression gradients. I just assumed that thin enough shells would solve the tension differential problem. Ultimately you really wouldn’t want rods per se, attached to the center shaft, but twice as long fibers, arranged to closely approach the center shaft so that their tension is shared only by their mid-strand material, and only rotationally coupled to the shaft otherwise. This would also allow the pancakes of inertial storage material to be mass produced and mass-tested more easily. Attaching them to a square cross section shaft seems like the optimal way to couple their inertial potential to the otherwise inert device mechanical shell. GoatGuy
⊕1 … I like your analysis. I definitely took a different path, calculus wise. I decided to evaluate the net vector force of dM (small masses, approaching lim→0) at geometrically small angles around a unit circle. Choose your numbers, but I chose at first 10. The whole mass of the rectangular cross-section shell is divided into 10 parts. Each is abstracted to 10 points of ¹⁄₁₀ the whole mass. The angle between each is ³⁶⁰⁄₁₀ → 36° or 2π/10 → π/5 radians. With a little trig, one goes on to compute over a full 10 point circle that each tension-vector at 36° (on either side of A point) is bearing ½ the outward centripetal force created by the spiing point dM mass. Iterating over 10, 30, 100, 300, … to see what the limit is, it turns out to converge quite rapidly. I ended up using 10,000 as the limit divisor, because the resulting tensile force was within 0.0001% of the higher implied limit. Good enough is good enough. However, figuring the force this way, I noted that the tensile force vector is shared by both neighbor particles and vice-versa. It is nicely balanced. Thus, redoing my original calculations a bit, these are the results for a material of ρ=1800 kg/m³ and a yield modulus of 54 GPa (divided by 2 for working tension):As fibers (rods) = 5,400 MPa⋅kg/kWhAs cylinder = 12,960 MPa⋅kg/kWhratio = cylinder / fiber → 2.4Obviously I could still be in error calculus wise, but I don’t quite think so. For me, these results confirm that old (very old) SciAm finding that spiing fibers, arranged radially on a spiing shaft axis, overlapped near the shaft to for a thicker hub and thier extended disk, delivers the highest rotational inertia for the same material tensile stress at working speed. Just saying,GoatGuy
Yep… I too read that SciAm article, what 25, 30 years ago?
⊕1 … I go to sleep, wake up, and find I’ve not only been well quoted in an NBF article, but bright people are taking on the math quantitatively! Happy days! GoatGuy
⊕1 for yor last sentence. Inertial energy storage is an EXCELLENT way to power railguns, and their far more benign cousins, the “mass launchers”. GoatGuy
Agreed: there ARE useful purposes for ultra-performance flywheel materials, to be sure. Clearly not “Earth to LEO” for all the reasons I outlined, but perhaps when materials science breaks 100 GPa working cyclic strength of fiber materials, there will be a lot of utility in deploying them in centripetal energy storage areas. Just saying,GoatGuy
Like GoatGuy I doubt that a flywheel powered rocket is useful, but flywheels for storing power for when a satellite goes into the shadow of a planet, or for power for a moonbase at night it seems useful.For powering a vehicle on a planet with no free oxygen, it is probably the best option. If the axis is vertical a failure can have the debris directed away from occupants of the vehicle.
I neglected the tension-compression gradients. I just assumed that thin enough shells would solve the tension differential problem.
Ultimately you really wouldn’t want rods per se, attached to the center shaft, but twice as long fibers, arranged to closely approach the center shaft so that their tension is shared only by their mid-strand material, and only rotationally coupled to the shaft otherwise.
This would also allow the pancakes of inertial storage material to be mass produced and mass-tested more easily. Attaching them to a square cross section shaft seems like the optimal way to couple their inertial potential to the otherwise inert device mechanical shell.
GoatGuy
⊕1 … I like your analysis.
I definitely took a different path, calculus wise. I decided to evaluate the net vector force of dM (small masses, approaching lim→0) at geometrically small angles around a unit circle. Choose your numbers, but I chose at first 10. The whole mass of the rectangular cross-section shell is divided into 10 parts. Each is abstracted to 10 points of ¹⁄₁₀ the whole mass. The angle between each is ³⁶⁰⁄₁₀ → 36° or 2π/10 → π/5 radians. With a little trig, one goes on to compute over a full 10 point circle that each tension-vector at 36° (on either side of A point) is bearing ½ the outward centripetal force created by the spinning point dM mass.
Iterating over 10, 30, 100, 300, … to see what the limit is, it turns out to converge quite rapidly. I ended up using 10,000 as the limit divisor, because the resulting tensile force was within 0.0001% of the higher implied limit. Good enough is good enough.
However, figuring the force this way, I noted that the tensile force vector is shared by both neighbor particles and vice-versa. It is nicely balanced.
Thus, redoing my original calculations a bit, these are the results for a material of ρ=1800 kg/m³ and a yield modulus of 54 GPa (divided by 2 for working tension):
As fibers (rods) = 5,400 MPa⋅kg/kWh
As cylinder = 12,960 MPa⋅kg/kWh
ratio = cylinder / fiber → 2.4
Obviously I could still be in error calculus wise, but I don’t quite think so. For me, these results confirm that old (very old) SciAm finding that spinning fibers, arranged radially on a spinning shaft axis, overlapped near the shaft to for a thicker hub and thinner extended disk, delivers the highest rotational inertia for the same material tensile stress at working speed.
Just saying,
GoatGuy
Yep… I too read that SciAm article, what 25, 30 years ago?
⊕1 … I go to sleep, wake up, and find I’ve not only been well quoted in an NBF article, but bright people are taking on the math quantitatively! Happy days! GoatGuy
⊕1 for yor last sentence. Inertial energy storage is an EXCELLENT way to power railguns, and their far more benign cousins, the “mass launchers”. GoatGuy
Agreed: there ARE useful purposes for ultra-performance flywheel materials, to be sure.
Clearly not “Earth to LEO” for all the reasons I outlined, but perhaps when materials science breaks 100 GPa working cyclic strength of fiber materials, there will be a lot of utility in deploying them in centripetal energy storage areas.
Just saying,
GoatGuy
Mythbusters made a machine gun that worked like that.
Like GoatGuy I doubt that a flywheel powered rocket is useful, but flywheels for storing power for when a satellite goes into the shadow of a planet, or for power for a moonbase at night it seems useful.
For powering a vehicle on a planet with no free oxygen, it is probably the best option. If the axis is vertical a failure can have the debris directed away from occupants of the vehicle.
not sure how to drive a rocket with a flyweel, but if it is possible, then there is also the option to just take the risk of it to explode (a havier launchpad), in worst case, it just wont lift off.It might be more interesting to use it for an (cyclic) electric railgun to space
In my analysis I neglected the interaction between volume elements of the flywheel, to simplify the math. The centrifugal force is proportional to the distance from the axis, so outer layers feel more centrifugal force than ier layers. If the flywheel is held together by hoop forces (fibers laid circumferentially), then I think that assumption of non-interaction (aka volume element independence) is correct, and the flywheel can be treated as an layered onion of independent thin shells, which gives my result. In this case, the hub and axis aren’t there for structural integrity, but only to transfer the rotation and keep the flywheel in position.However, if the flywheel is held together by radial forces, then the outer layers pull on the ier layers, which in turn pull on the axis. In that case, my assumption is incorrect, and I would expect to get the tapered geometry as the correct answer.The standard flywheel design is the former. The question is, which of these is better energetically. I’m too short on time right now to do the math for the 2nd design.
IIRC, an article in Scientific American, (Back when it was still readable.) on the topic demonstrated that the optimal shape was thick in the center and tapered to the rim, assuming you want to load the whole thing to maximum stress.
Yes, but as I understood from his multitude of posts, he did consider other geometries, and came to the conclusion that a rod shaped flywheel (or something equivalent to a collection of such rods) is optimal. Which is wrong, apparently.
Hmm.. I was imagining the mass flying off radially, because that’s the direction of the load. But you’re right, the velocity is tangential, and once the material breaks off, there are no more forces on it. So it’ll fly off tangentially. Good points.
Just checked my result against the table that Brian posted in /2018/10/cnt-bundle-material-for-flywheels-40-times-better-than-batteries html. Every row in that table fits my formula exactly:Esp [J/kg] = 0.5 * Ysp [N*m/kg]or in Wh:Esp [Wh/kg] = 0.5 * Ysp [N*m/kg] / 3600 [J/Wh]This confirms my analysis further.
Explicitly, he was using a rod shaped flywheel, not an optimized shape. Not so much an “error”, as a failure to optimize.
Well, to answer that last question first, if you ablate material off the rim of a flywheel, it goes off on a tangent, and the flywheel goes in the opposite direction. I suppose you can have a hole in the housing for the exhaust to exit through, since it’s going to be a controlled disassembly of the flywheel.And if you want a decent ISP, you are going to ablate the material off the rim, because that’s where it’s traveling fastest.Thinking back on an old proposal, though, if your flywheel had plumbing in it, and fed a liquid or powder in at the center, and released it at the rim in a controlled maer, you could get the same effect, only still having your flywheel at the end. This was one of the proposals to use ground up asteroid as reaction mass, it was called a “slinger”; A pair of them could convert electricity into thrust very efficiently using any mass you could feed through them, just using an electric motor at the hub.
For nanotechnology applications, theoretically yes. Assuming the circumferential tensile strength of a single CNT is that of perfect graphene, 130 GPa, and density is ~1500 kg/m^3, then a rough estimate of the energy density is 0.5 * 130e9 / 1500 = 43e6 [J/kg] = 43 [MJ/kg]. If the tensile strength is closer to the theoretical strength of CNTs, 63 GPa, the energy density is 21 [MJ/kg]. Divide by some factor for safety margin, housing, and support systems, so practical value is closer to 5-10 MJ/kg.Use a multiwalled nanotube with the outer layers serving as bearing and housing, and the ier ones as the flywheel. You’d need to functionalize the ier tubes to allow some way to transfer energy to and from the nanoflywheel.
Even if it was economic and you weren’t joking, according to my analysis, stored energy is proportional to distance from the axis. So ablating the outer rim is a bad engineering choice. Plus you need a housing for safety, so where’s the ablated material going to go?
Based on GoatGuy’s calculus, he concluded in the previous articles that a cylindrical shape is the wrong shape for a flywheel. But every flywheel article I’ve found suggests the opposite. There’s even work on hubless flywheels with magnetic bearings, to eliminate the less useful weight and cost of the hub. You’d think that if that was the wrong shape, someone would’ve picked up on that.My own analysis supports the contrary conclusion that a cylindrical shell is the best shape. I’ve posted the full analysis in NBF /2018/10/cnt-bundle-material-for-flywheels-40-times-better-than-batteries html. In summary:Neglecting interaction between volume elements, for a volume element dV = dA*dr at distance r from the axis and angular velocity w, under pure radial load:Esp = 0.5*(w*r)^2 (specific energy, [J/kg])Ysp = Lsp = w^2*r*dr (specific radial load, directly comparable to specific yield strength, [N*m/kg])Esp/Ysp = 0.5*r/dr ([J/N*m] aka unitless)dr is constant, so for any given specific yield strength Ysp, the max specific energy is proportional to the distance from the axis, r.If we assume the fibers arranged in a circumferential loop, with the loads translated to tangential loads, then using the formula for hoop stress of a thin-walled cylinder, we get:Ysp = Lsp = (w*r)^2 (specific tangential load, directly comparable to specific yield strength, [N*m/kg])Esp/Ysp = 0.5 ([J/N*m] aka unitless)Under pure hoop load, both load and energy scale quadratically with the distance from the axis, so the max specific energy depends only on the specific yield strength, regardless of mass distribution.These results also fit Adam Crawl’s energy density value, if we allow for an extra factor of 2 for safety margin and housing:Y = 54 [GPa] = 5.4e10 [Pa]d = 1800 [kg/m^3]Ysp = Y/d = 3e7 [N*m/kg]Esp = 0.5*Ysp (my result) * 0.5 (safety and housing) = 0.5 * 0.5 * 3e7 [N*m/kg] = 7.5e6 [N*m/kg] = 7.5 [MJ/kg]Furthermore, GoatGuy’s result of 10.8 [L*MPa/kWh] doesn’t seem to match published performance figures (unless I calculated wrong). These results lead me to believe there is an error in GoatGuy’s analysis.
Mythbusters made a machine gun that worked like that.
Makes me wonder if there would be a way to store energy by spiing individual nanotube/nanorods on their axis. Too lazy to do the math -_-
not sure how to drive a rocket with a flyweel, but if it is possible, then there is also the option to just take the risk of it to explode (a havier launchpad), in worst case, it just wont lift off.
It might be more interesting to use it for an (cyclic) electric railgun to space
In my analysis I neglected the interaction between volume elements of the flywheel, to simplify the math. The centrifugal force is proportional to the distance from the axis, so outer layers feel more centrifugal force than inner layers. If the flywheel is held together by hoop forces (fibers laid circumferentially), then I think that assumption of non-interaction (aka volume element independence) is correct, and the flywheel can be treated as an layered onion of independent thin shells, which gives my result. In this case, the hub and axis aren’t there for structural integrity, but only to transfer the rotation and keep the flywheel in position.
However, if the flywheel is held together by radial forces, then the outer layers pull on the inner layers, which in turn pull on the axis. In that case, my assumption is incorrect, and I would expect to get the tapered geometry as the correct answer.
The standard flywheel design is the former. The question is, which of these is better energetically. I’m too short on time right now to do the math for the 2nd design.
IIRC, an article in Scientific American, (Back when it was still readable.) on the topic demonstrated that the optimal shape was thick in the center and tapered to the rim, assuming you want to load the whole thing to maximum stress.
Yes, but as I understood from his multitude of posts, he did consider other geometries, and came to the conclusion that a rod shaped flywheel (or something equivalent to a collection of such rods) is optimal. Which is wrong, apparently.
Hmm.. I was imagining the mass flying off radially, because that’s the direction of the load. But you’re right, the velocity is tangential, and once the material breaks off, there are no more forces on it. So it’ll fly off tangentially. Good points.
Just checked my result against the table that Brian posted in /2018/10/cnt-bundle-material-for-flywheels-40-times-better-than-batteries html. Every row in that table fits my formula exactly:
Esp [J/kg] = 0.5 * Ysp [N*m/kg]
or in Wh:
Esp [Wh/kg] = 0.5 * Ysp [N*m/kg] / 3600 [J/Wh]
This confirms my analysis further.
Explicitly, he was using a rod shaped flywheel, not an optimized shape. Not so much an “error”, as a failure to optimize.
Well, to answer that last question first, if you ablate material off the rim of a flywheel, it goes off on a tangent, and the flywheel goes in the opposite direction. I suppose you can have a hole in the housing for the exhaust to exit through, since it’s going to be a controlled disassembly of the flywheel.
And if you want a decent ISP, you are going to ablate the material off the rim, because that’s where it’s traveling fastest.
Thinking back on an old proposal, though, if your flywheel had plumbing in it, and fed a liquid or powder in at the center, and released it at the rim in a controlled manner, you could get the same effect, only still having your flywheel at the end. This was one of the proposals to use ground up asteroid as reaction mass, it was called a “slinger”; A pair of them could convert electricity into thrust very efficiently using any mass you could feed through them, just using an electric motor at the hub.
It’s the total energy, not the energy/kg that’s off concern.A 1 kg flywheel with 10 kWh shouldn’t be more dangerous than a 50 kg supercapacitor, with 10 kWh. Just lighter and easier to move around. And because it’s smaller it would be easier to enclose in a blast shield.
2500 MPa steel can cost less than $1/kg. This steel may be competitive to be a flywheel rotor material.
A 10 kWh/kg flywheel should be safe when installed several metres below ground level. Are there other economical safe containments?
For nanotechnology applications, theoretically yes. Assuming the circumferential tensile strength of a single CNT is that of perfect graphene, 130 GPa, and density is ~1500 kg/m^3, then a rough estimate of the energy density is 0.5 * 130e9 / 1500 = 43e6 [J/kg] = 43 [MJ/kg]. If the tensile strength is closer to the theoretical strength of CNTs, 63 GPa, the energy density is 21 [MJ/kg]. Divide by some factor for safety margin, housing, and support systems, so practical value is closer to 5-10 MJ/kg.
Use a multiwalled nanotube with the outer layers serving as bearing and housing, and the inner ones as the flywheel. You’d need to functionalize the inner tubes to allow some way to transfer energy to and from the nanoflywheel.
So flywheels could be the next superbattery, but still not enough to have battery powered rockets.They still allow much better battery powered vehicles. Especially for the users who are brave enough to take off the “deadweight” shatter shields around the flywheel and run them “naked” and 500 kg lighter.Who remembers the cookie cutter nanobots from The Diamond Age?
Even if it was economic and you weren’t joking, according to my analysis, stored energy is proportional to distance from the axis. So ablating the outer rim is a bad engineering choice. Plus you need a housing for safety, so where’s the ablated material going to go?
Based on GoatGuy’s calculus, he concluded in the previous articles that a cylindrical shape is the wrong shape for a flywheel. But every flywheel article I’ve found suggests the opposite. There’s even work on hubless flywheels with magnetic bearings, to eliminate the less useful weight and cost of the hub. You’d think that if that was the wrong shape, someone would’ve picked up on that.
My own analysis supports the contrary conclusion that a cylindrical shell is the best shape. I’ve posted the full analysis in NBF /2018/10/cnt-bundle-material-for-flywheels-40-times-better-than-batteries html. In summary:
Neglecting interaction between volume elements, for a volume element dV = dA*dr at distance r from the axis and angular velocity w, under pure radial load:
Esp = 0.5*(w*r)^2 (specific energy, [J/kg])
Ysp = Lsp = w^2*r*dr (specific radial load, directly comparable to specific yield strength, [N*m/kg])
Esp/Ysp = 0.5*r/dr ([J/N*m] aka unitless)
dr is constant, so for any given specific yield strength Ysp, the max specific energy is proportional to the distance from the axis, r.
If we assume the fibers arranged in a circumferential loop, with the loads translated to tangential loads, then using the formula for hoop stress of a thin-walled cylinder, we get:
Ysp = Lsp = (w*r)^2 (specific tangential load, directly comparable to specific yield strength, [N*m/kg])
Esp/Ysp = 0.5 ([J/N*m] aka unitless)
Under pure hoop load, both load and energy scale quadratically with the distance from the axis, so the max specific energy depends only on the specific yield strength, regardless of mass distribution.
These results also fit Adam Crawl’s energy density value, if we allow for an extra factor of 2 for safety margin and housing:
Y = 54 [GPa] = 5.4e10 [Pa]
d = 1800 [kg/m^3]
Ysp = Y/d = 3e7 [N*m/kg]
Esp = 0.5*Ysp (my result) * 0.5 (safety and housing) = 0.5 * 0.5 * 3e7 [N*m/kg] = 7.5e6 [N*m/kg] = 7.5 [MJ/kg]
Furthermore, GoatGuy’s result of 10.8 [L*MPa/kWh] doesn’t seem to match published performance figures (unless I calculated wrong). These results lead me to believe there is an error in GoatGuy’s analysis.
Just joking about that, of course. While it would work, it could never be economic.
Ah, if you’ve got this high energy flywheel, couldn’t you ablate the rim at just the right point to provide controlled thrust? Providing relatively direct transformation of stored energy into thrust, and lowering the mass of the vehicle as the flywheels were exhausted?
Lots of people are working on carbon nanotube materials, China has no exclusivity, and it is not destined to control the world as many Chinese people believe from the simple reason that other nations do not want to be controlled by oppressive China.
Makes me wonder if there would be a way to store energy by spinning individual nanotube/nanorods on their axis. Too lazy to do the math -_-
It’s the total energy, not the energy/kg that’s off concern.
A 1 kg flywheel with 10 kWh shouldn’t be more dangerous than a 50 kg supercapacitor, with 10 kWh. Just lighter and easier to move around. And because it’s smaller it would be easier to enclose in a blast shield.
2500 MPa steel can cost less than $1/kg. This steel may be competitive to be a flywheel rotor material.
A 10 kWh/kg flywheel should be safe when installed several metres below ground level. Are there other economical safe containments?
So flywheels could be the next superbattery, but still not enough to have battery powered rockets.
They still allow much better battery powered vehicles. Especially for the users who are brave enough to take off the “deadweight” shatter shields around the flywheel and run them “naked” and 500 kg lighter.
Who remembers the cookie cutter nanobots from The Diamond Age?
Just joking about that, of course. While it would work, it could never be economic.
Ah, if you’ve got this high energy flywheel, couldn’t you ablate the rim at just the right point to provide controlled thrust? Providing relatively direct transformation of stored energy into thrust, and lowering the mass of the vehicle as the flywheels were exhausted?
Lots of people are working on carbon nanotube materials, China has no exclusivity, and it is not destined to control the world as many Chinese people believe from the simple reason that other nations do not want to be controlled by oppressive China.