Peak laser intensity demonstrations have occurred on specific Nd:glass-based lasers:
* the Vulcan PW in the UK at 1 × 10^21 W/cm2 (2004);
* the Ti:sapphirebased HERCULES laser at the University of Michigan, USA at 1 × 10^22 W/cm2 (2004)
* J-KAREN-P in Japan at 1 × 10^22 W/cm2 (2018)
* record intensity of 5.5 × 10^22 W/cm2 was demonstrated at the CoReLS laser (2019)
Even the highest-peak-power laser systems (10 PW and beyond) proposed or already in commissioning make no exception to this trend and largely predict intensities of only up to 10^23 W/cm2
(notably L4-ELI, EP-OPAL , SULF and SEL).
A fundamental physics or engineering limit is not clear; however, material challenges such as imperfect diffraction gratings, optics and gain materials reduce the overall laser focusability in time and space.
The steady ascent of Ti:sapphire, OPCPA and Nd:glass technologies upward in peak power has, with the construction of several ten to multi-tens of petawatt systems, nearly reached the ∼100 PW limit of metre-scale gold diffraction gratings.
The continued progress of ultra-intense CPA lasers must take a multi-beam approach. Non-CPA Nd:glass fusion lasers have taken this approach for decades, which is most dramatically demonstrated with the 192-beam NIF laser. This could take the form of either incoherent or coherent beam combination of ∼10 PW scale beams. Indeed, the Shanghai SEL facility is already taking that approach to reach the 100 PW scale by combining four multi-tens of petawatt beams.
Ultra-high-power development
The drive to deliver ever-increasing powers is driven largely by a desire to achieve ever-increasing intensities to target to achieve focused intensities over 10^23 W/cm2. At these focused intensities new regimes of physics could be realized. Various techniques could be used to scale lasers to exawatt class facilities. Three techniques are
• 100 PW OPCPA systems
• Alternative 100 PW schemes
• Plasma amplifiers.
The primary challenges being developed for 100 PW OPCPA ranked by difficulty are:
(1) Advanced gratings; large-aperture DKDP; specialized optical coatings for large-aperture mirrors.
(2) Wavefront control, adaptive optics, and two-stage focusing to maximize focused intensity.
(3) Ultra-short-pulse laser diagnostics and broadband dispersion control.
(4) Laser subsystem development including broadband front end and OPA gain adjustment.
A common feature of the exawatt scale (over 200 PW) facilities is a requirement for coherent pulse combination as a means of generating sufficient power, due to the constraints of individual beam delivery.
The original concept of ELI was for another facility to use a coherent superposition of up to ten 20 PW beamlines to produce 200 PW to target. The facility is still on the agenda but is currently unfunded. All three of the existing pillars are contributing to high-power laser development or nonlinear conversion techniques to generate the baseline technologies to approach the 200 PW level and ultimately
generate focused intensities over 10^25 W/cm2. In 2011, the international institute IZEST (International Institute for Zettawatt-Exawatt Science and Technology) was created to provide scientific and organizational support of projects aimed at developing exawatt power lasers and their applications.
The next decade will see a dramatic increase in development work across the globe for the delivery of over 100 PW systems, together with the SEL project expected to come online in
2023. These facilities will open up a new regime in discovery science as focused intensities in excess of 10^23 W/cm2 could be realized. To achieve 100 PW operation of future systems with modest energies, researchers are also endeavoring to reduce the pulse width of these lasers towards the single cycle limit.
Compression after Compressor Approach (CafCA)
The main limitation of laser power is the damage threshold and physical size of diffraction gratings. It is not possible to increase the pulse energy after grating compression, but laser power may be increased by pulse shortening. The technique described here is called Compression after Compressor Approach (CafCA) and is based on spectral broadening by selfphase modulation (SPM) in nonlinear plates and eliminating the spectral phase through chirped mirror(s). This idea has been successfully used in mJ pulse energy systems since the 1980s, but power scaling was limited by the aperture of gas-filled capillaries and self-focusing. CafCA could drastically increase the power of any laser from terawatts to tens of petawatts.
In recent years CafCA was demonstrated with crystals, glasses, or plastics, in which the most powerful experiment to date was carried out where a 100- mm-diameter 5.5 J pulse was compressed from 57 fs to 22 fs. This got around the capillary problem.
Nexawatt
Currently, scaling of petawatt lasers to higher pulse energy and peak powers is limited by several things: intensity-dependent damage thresholds of postcompression and final focusing optics, insufficient stretched pulse durations needed to avoid damage to amplifier and transport optics, and a lack of pre-amplifier bandwidth to support shorter pulse durations.
The Nexawatt concept proposed at the Lawrence Livermore National Laboratory, US relies on extracting the full potential of the disc amplifiers of a NIF or NIF-like beamline (∼25 kJ) but using a novel compressor design to confine this energy to a 100 fs pulse, producing pulses with peak powers of over 200 PW. This could be achieved using a typical four-grating compressor; however, two of the gratings would require apertures of 4.5 meters. A six-grating compressor design is proposed utilizing gratings with a more achievable 2-meter aperture manufactured by stitched lithographic exposure.
Damage to final optics is avoided by increasing beam area via splitting the beam prior to compression and then coherently recombining the beams prior to focusing, where peak intensities of 10^26 W/cm2 are anticipated.
Plasma Amplifiers
Novel laser amplification techniques such as OPCPA or diode-pumped solid-state lasers are threatening to overtake even the theoretical predictions for Raman amplification for near-infrared laser pulses. Thus, Raman amplification needs to find new niches where these competing techniques are not strong:
(i) scalability to other wavelengths;
(ii) scalability of signal pulse parameters with pump intensity and duration; and
(iii) amplification of higher-order laser modes, rather than just Gaussian
High Average Power
Getting both high-peak and high-average powers simultaneously is therefore becoming increasingly important. The first particle acceleration demonstrations relied on petawatt laser systems with relatively low repetition rates (1 Hz) like BELLA at LNBL, USA, that were energized by multiple, aperture-combined flashlamp-pumped Nd:YAG lasers. The HAPLS laser developed by LLNL for ELI-Beamlines is the highest average power diodepumped petawatt laser capable of delivering up to 300 Watts of average power for user experiments. However, future particle accelerators would require the laser to operate at repetition rates orders of magnitude higher to reach the hundreds of kW average power needed.
Techniques which are making significant advances in the development of systems to deliver high-average-power capabilities:
• HAP gas-cooled architectures
• Scaling petawatt class lasers beyond 10 kW
• Cryo-HAP laser development
• Coherent beam combining
• Time-domain pulse combining
• Temperature-insensitive OPCPA.
SOURCES- High Power Laser Science and Engineering, (2019), Vol. 7, e54, 54 pages – Petawatt and exawatt class lasers worldwide
Written By Brian Wang, Nextbigfuture.com
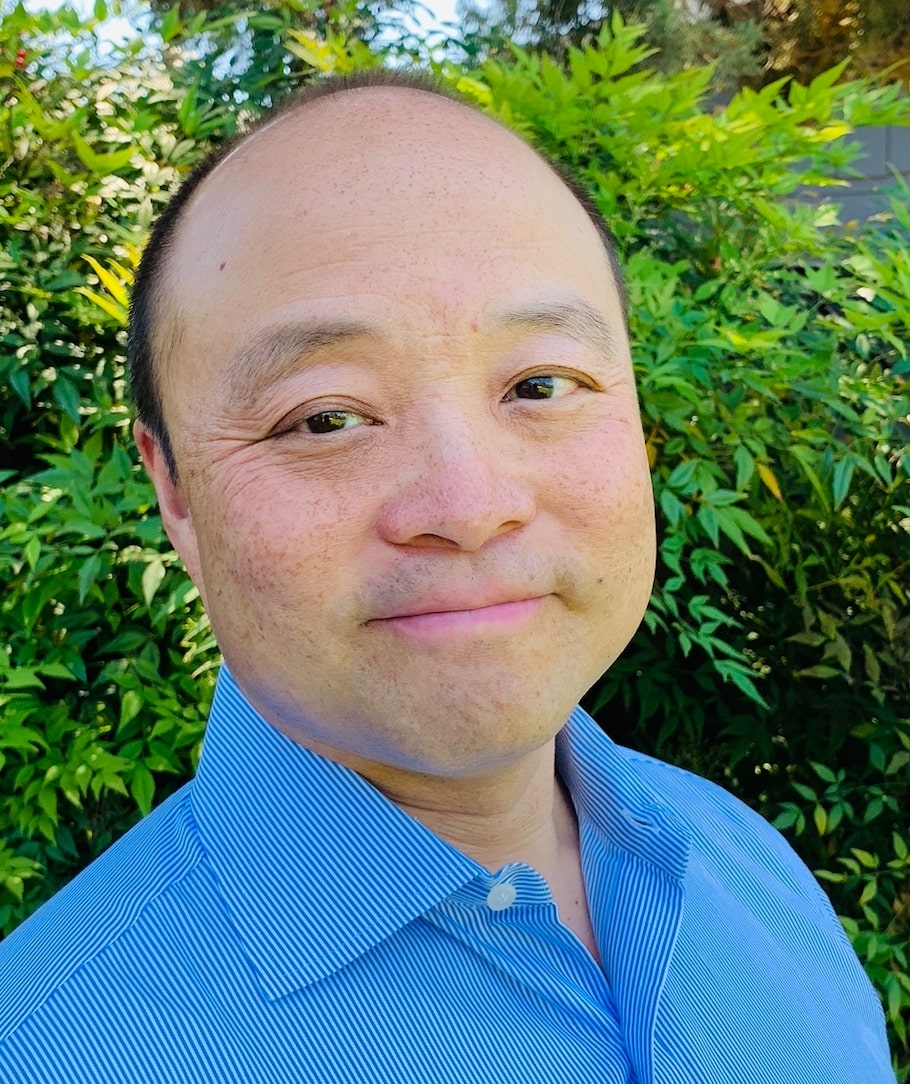
Brian Wang is a Futurist Thought Leader and a popular Science blogger with 1 million readers per month. His blog Nextbigfuture.com is ranked #1 Science News Blog. It covers many disruptive technology and trends including Space, Robotics, Artificial Intelligence, Medicine, Anti-aging Biotechnology, and Nanotechnology.
Known for identifying cutting edge technologies, he is currently a Co-Founder of a startup and fundraiser for high potential early-stage companies. He is the Head of Research for Allocations for deep technology investments and an Angel Investor at Space Angels.
A frequent speaker at corporations, he has been a TEDx speaker, a Singularity University speaker and guest at numerous interviews for radio and podcasts. He is open to public speaking and advising engagements.
Since nobody else is saying it, the ability to destroy a planet is insignificant next to the power of the Force.
Yea, I was talking about the lasers in this article. High power, not so much high energy.
Btw, I’m not getting reply notifications by email recently. Is it just me, or did something break?
To be pedantic, a supernova also has high energy. High power. High force. High intensity. High mass. High everything.
But the lasers (under discussion) do not.
I am suprised there aren’t more larger teams researching approach to fusion using lasers.
They could build more smaller prototypes- the proof of concept and research/develop some side technologies even if the lasers aren’t there yet. You could do a lot even if some pieces of the puzzle are missing and get them later. Laser tech is improving quite fast.
The benefits if we get commercial fusion reactors are so huge it is unreasonable to dismiss it with simple sentence – which is mentality against progress -“It can’t be done”.
Very harm ful thinking, except some exceptions. The correct mentality would be how can we make it work? How could we solve that problem? That would be much better approach.
I guess coal/oil lobby wouldn’t like it and would work to cut funding off.
I just happened to read about that yesterday. Apparently CR production has to do with how protons interact with the magnetic field in a supernova. They get bounced around a few times, each time picking more energy, until they’re no longer contained. Kind of like hail in a storm cloud. Or something like that. Googling “cosmic rays supernova” will give you more info.
(Btw, here we’re talking high power levels, not so much high energy. The timescales are very short.)
Whatever process makes cosmic rays ought to have destroyed the universe long ago, if such energy levels were of any danger to it.
I don’t think this exceeds the intensities experience in things like supernovas and black hole collisions, so if the universe were to break it would already happen.
But I could be wrong.
Note that when nuclear bombs were first developed, there was some concern that the atmosphere itself could be subject to a chain reaction fusion reaction, and that hence letting of a nuke anywhere could destroy the world. It was also worried that lithium 7 could fuse and so give a much bigger explosion than planned.
So they ran through the calculations and showed that both sets of worries were unfounded, and neither reaction could run away.
It turned out that one of the calculations was wrong. But not the one that would have resulted in the world exploding.
I hate people with a vengeance
Will this break the universe?
Nice to see the advancement in lasers. For fusion we will need powerful but efficient lasers with high repetition rates. There need to be specific research on that kind of laser.
The Zetawatt graph/Table and below graphs are not in the paper you cite as source.Where are they come from?
“lazer”