Researchers have now taken a step toward that vision of molecular systems that repair cells inside the human body. Molecular repair built from a patient’s own cells will eventually ferret out cancer, repair injured tissue, and even remove plaque from blood vessels.
They have gotten tracheal cells to form coordinated groups called organoids that can propel themselves with tiny appendages. When added to wounded neurons in the lab, these anthrobots helped neurons repair themselves.
These organoids can help with monitoring inside the body, delivering drugs and improving repair at the cellular level. These are living versions of the vision of nanobots. Advanced versions of these systems could accelerate healing of neurons, nerves and the spinal cord and many other tissues. The neuron, nerve and spinal cord repair would be critical for the brain repair, brain swapping and new body growth proposal for a form of immortality.
Michael Levin, a developmental biologist at Tufts University and leader of the new study, made his first living robots 4 years ago. He and his colleagues stitched together embryonic heart and skin cells from the African clawed frog to create an organoid with cilia, tiny hairs that move back and forth, enabling it to crawl and even swim. Levin demonstrated that cells can be coached to do something they would never have done on their own.
In the new study, Levin’s graduate student, Gizem Gumuskaya, started with cells that line the adult human trachea. These cells also sport cilia, and the researchers hoped to harness the appendages as tiny oars to power and move the organoid.
Gumuskaya put individual tracheal cells in a 3D scaffolding, made from rat tissue, which resembles the environment in the human trachea. After 2 weeks, the cells multiplied and formed tiny spheres, but their cilia were inside these spheres and thus useless for locomotion. So the researchers immersed the cells in a special bath, whose liquid properties prompted them to turn their cilia out.
The resulting organoids differ in size and shape, despite having the same DNA. They can be round or oblong and consist of between 100 to 1000 cells. (The largest of them is about the size of a poppy seed.) Their cilia may be spread across their entire outer surface or clustered in discrete patches.
To get a sense whether these anthrobots might interact with human tissue, Levin’s team used a well-established method to assess wound healing in the nervous system. Researchers grow a sheet of nerve cells in lab dish and wound them by scratching the sheet.
Gumuskaya added clusters of anthrobots to this lab dish, the organoids moved onto and down the scratch. Then, to her surprise, a bridge of nerve tissue formed across the scratch where the anthrobots settled, healing the injury. Nonliving substances, such as starch or silicone, had no such effect on the scratch, Levin says. He thinks that, as living tissue, the anthrobots helped the nerve cells on one side of the scratch sense where the other side was so they could initiate new growth.
The work was partially funded by a biotech start up called Astonishing Labs, which aims to harness the technology to treat neurological disease and nerve and spinal cord injuries. The company’s managing partner Thomas Cirrito also envisions applying anthrobots to help heal burns.
Ren and Walter Finkbeiner, a pathologist emeritus at the University of California, San Franciso, see potential to use the anthrobots as screening tools for pollutants, new drugs, and carcinogens. Scientists can easily monitor whether the organoids are still alive and healthy based on whether they’re still moving.
Researchers can enhance these organoids’ potential by modifying their genomes for desired functions, such as delivering cancer drugs. Levin notes that anthrobot delivery vehicles may be less toxic than existing drugs and less likely to aggravate the immune system than implantable devices. He even envisions harnessing the anthrobots’ potential sensing and memory capabilities—which drugs and implantable devices lack—to monitor the body’s health status.
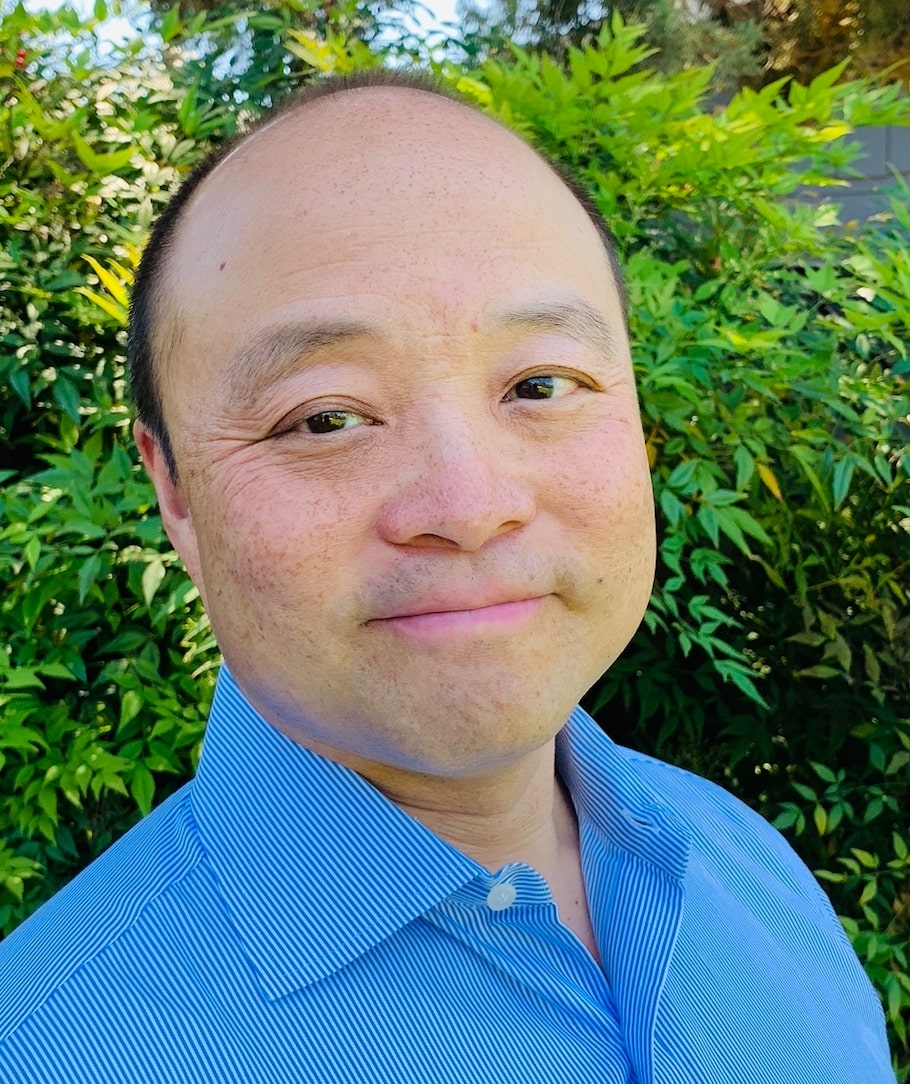
Brian Wang is a Futurist Thought Leader and a popular Science blogger with 1 million readers per month. His blog Nextbigfuture.com is ranked #1 Science News Blog. It covers many disruptive technology and trends including Space, Robotics, Artificial Intelligence, Medicine, Anti-aging Biotechnology, and Nanotechnology.
Known for identifying cutting edge technologies, he is currently a Co-Founder of a startup and fundraiser for high potential early-stage companies. He is the Head of Research for Allocations for deep technology investments and an Angel Investor at Space Angels.
A frequent speaker at corporations, he has been a TEDx speaker, a Singularity University speaker and guest at numerous interviews for radio and podcasts. He is open to public speaking and advising engagements.